Thank you for visiting nature.com. You are using a browser version with limited support for CSS. To obtain the best experience, we recommend you use a more up to date browser (or turn off compatibility mode in Internet Explorer). In the meantime, to ensure continued support, we are displaying the site without styles and JavaScript.
- View all journals
- Explore content
- About the journal
- Publish with us
- Sign up for alerts
- Open access
- Published: 17 October 2018

Spatial trends in United States tornado frequency
- Vittorio A. Gensini ORCID: orcid.org/0000-0001-6362-9197 1 &
- Harold E. Brooks 2
npj Climate and Atmospheric Science volume 1 , Article number: 38 ( 2018 ) Cite this article
61k Accesses
100 Citations
1954 Altmetric
Metrics details
- Climate change
- Climate sciences
Severe thunderstorms accompanied by tornadoes, hail, and damaging winds cause an average of 5.4 billion dollars of damage each year across the United States, and 10 billion-dollar events are no longer uncommon. This overall economic and casualty risk—with over 600 severe thunderstorm related deaths in 2011—has prompted public and scientific inquiries about the impact of climate change on tornadoes. We show that national annual frequencies of tornado reports have remained relatively constant, but significant spatially-varying temporal trends in tornado frequency have occurred since 1979. Negative tendencies of tornado occurrence have been noted in portions of the central and southern Great Plains, while robust positive trends have been documented in portions of the Midwest and Southeast United States. In addition, the significant tornado parameter is used as an environmental covariate to increase confidence in the tornado report results.
Similar content being viewed by others
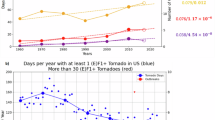
The regionality and seasonality of tornado trends in the United States

Explaining the trends and variability in the United States tornado records using climate teleconnections and shifts in observational practices
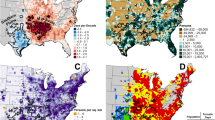
Changes in tornado risk and societal vulnerability leading to greater tornado impact potential
Introduction.
Recent trends in global and United States temperature have provoked questions about the impact on frequency, intensity, timing, and location of tornadoes. When removing many non-meteorological factors, it is shown that the annual frequency of United States tornadoes through the most reliable portions of the historical record has remained relatively constant. 1 , 2 , 3 , 4 The most notable trends in tornado frequency are associated with increasing annual variability and a recent tendency for more tornadoes on any given tornado day. 5 , 6 , 7 , 8 , 9 There has also been a measurable shift in the annual timing of tornado season, but it is currently unknown if this is due to rising global temperatures or natural variability. 10 , 11 Two recent studies show increased tornado frequency in the eastern United States and decreased activity generally in the central United States, but analyses were limited to epochs. 7 , 12 In addition, decreasing tornado frequency trends in the Great Plains and increasing trends in the Southeast are present in annual counts of E(F)1 + tornadoes. 13 Despite these changes, detecting spatial shifts in tornado frequency has proved challenging given the relatively small spatial and temporal scales associated with tornado-producing thunderstorms and the well-documented deficiencies accompanying the tornado reporting database. 1 The work herein seeks to examine spatial trends in tornado environments and tornado frequency at finer-scale resolutions in space and time.
These deficiencies have motivated researchers to focus on developing and analyzing atmospheric derived indices relating environmental conditions to tornado occurrence. Such indices are often used in an ingredients-based approach commonly utilized in operational weather forecasting. 14 The significant tornado parameter (STP) is one such index, originally designed as a statistical discriminator between non-tornado and significant ([E]F2 or greater using the Fujita damage scale) tornado environments. 15 STP is a commonly used operational weather forecasting index, but no research has used STP to examine the covariant relationship with aspects of the United States tornado report climatology.
A derived covariate, such as STP, is complementary to tornado reports for climatological studies. For example, it has been shown that variables like storm relative helicity and convective precipitation adequately represent United States monthly tornado frequency. 16 Reports are subject to a human reporting process, whereas environmental covariates allow for an objective model-derived climatology. One major limitation of the covariate methodology for tornadoes is the lack of accounting for a thunderstorm initiation metric. It is well documented that tornadoes occur in environments characteristic of adequate vertical wind shear, relatively high surface water vapor mixing ratios, low static stability, and directional change in the lowest 1-km of tropospheric wind. 17 , 18 Yet, even with a supportive atmosphere, thunderstorms may not form in the absence of an initial lifting mechanism needed to commence the convective process. The space and time scales of various lifting sources are often below the spatial scale of current reanalysis observation capabilities, and thus, not accounted for by most covariate environmental metrics. This often leads to an overestimation of favorable tornado environments and a lack of explanatory capability regarding observed tornado reports, especially when convective inhibition is present. 19
Recent increases in computational efficiency have led several studies to explicitly depict severe convective storms in convective permitting regional climate model configurations to evade the previously discussed environmental caveats. 20 , 21 , 22 , 23 , 24 , 25 These dynamical downscaling approaches (sometimes referred to as model telescoping) have proven reliable for the replication of spatial frequency and temporal timing of the annual peak of boreal severe weather activity. Potential changes in characteristics of late 21 st century severe weather activity have also been posited by these methods. However, due to the resource intensive nature of these approaches, long model-derived climatologies are often unfeasible and it is not yet clear if the explanatory capability is significantly better than environments to justify the resources necessary to conduct such simulations.
This research focuses on an environmental covariate approach to examine potential changes in United States tornado frequency. In particular, we begin by analyzing an aggregated covariate methodology in an attempt to explain the variance associated with monthly and annual United States tornado reports. We further examine the spatio-temporal changes in this covariate over the past ~40 years. This allows us to draw conclusions about the regional trends in frequency of tornado environments. We conclude by comparing the covariate trend analyses to actual tornado reporting trends to strengthen the presented results. Ultimately, our research highlights that trends in United States tornado frequency are regional in nature and this change in physical risk has implications for tornado exposure and vulnerability.
Despite the aforementioned environmental caveats, annual accumulated diurnal max STP is a statistically significant covariate to annual United States tornado reports (Fig. 1 ). This degree of explanatory power (44% of the variance in annual tornado counts is explained by accumulated diurnal maximum STP) suggests that STP may be used as a climatological proxy for annual tornado report counts. At the monthly scale, accumulated STP exhibits a seasonal trend in explanatory power. Monthly standardized anomalies of accumulated diurnal maximum STP for January, February, March, May, and December all explain roughly half of the variance associated with the standardized anomaly of monthly United States tornado counts (Fig. 2 ). August displays a minimum in variance explanation (9%), and it is speculated this is a function of the mesoscale nature of late boreal summer tornado environments and larger contributions to the monthly count climatologies from tropical storms and hurricanes. Standardized anomaly regression slopes reveal that monthly accumulated STP is a conservative estimator of monthly tornado counts, and this bias is greatest in the boreal summer (Fig. 2 ). The null hypothesis of, “Monthly accumulated STP does not explain statistically significant amounts of variance in monthly tornado counts” was rejected at the 0.01 significance level for all months except August. Thus, the STP covariate can be applied at the monthly scale, but caution should be used due to the seasonal changes in the coefficient of determination and level of statistical significance.
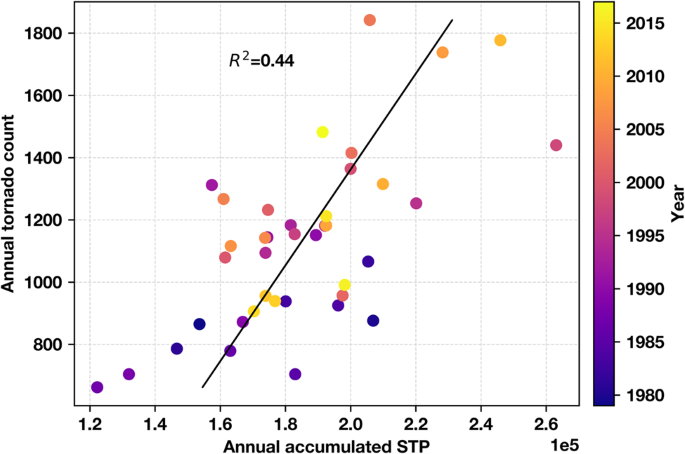
Scatter plot of United States annual tornado counts vs. United States annual accumulated significant tornado parameter (1979–2017) from NARR
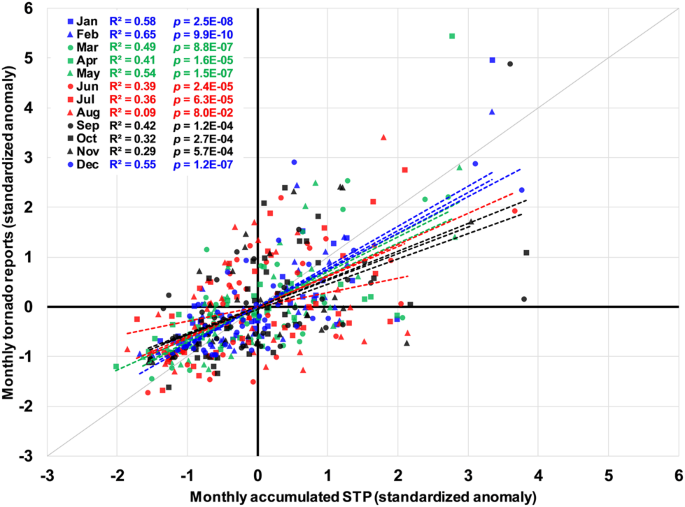
1979–2017 Unites States monthly tornado reports and accumulated diurnal maximum STP (expressed here as a standardized anomaly by month) from NARR. Regression r 2 and p values are listed next to their respective months
Examining annual tornado report counts and accumulated annual STP through time confirm this explanatory relationship and give further confidence in the use of STP as a climatological tornado report proxy (Fig. 3 ). Both tornado counts and STP indicate a slight positive trend through time over the analysis period, which starts at the beginning of the modern Geostationary Operational Environmental Satellite (GOES) era. Consistent tornado reporting data exists back to the early 1950s, but there are currently no datasets that allow for the space and time scales necessary to adequately retroactively compute STP back to 1950, and hence, we are limited here to the analysis period 1979–2017. Most of the increase in tornado reporting is observed from the late 1980s to the early 2000s, with a steady or even declining trend thereafter which is consistent with previous research. 3
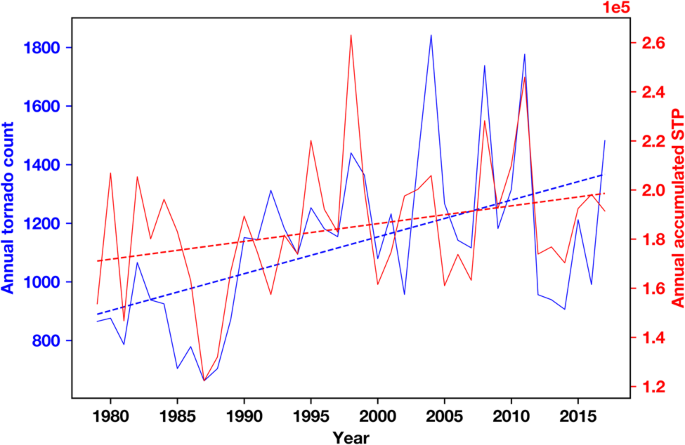
Interannual variability of United States tornado reports (blue; axis left) and the United States annual sum of daily max significant tornado parameter (red; axis right) from NARR
Of greatest interest here is the potential for shifts in the spatial location of tornado frequency. Research has identified evidence of a “Dixie Alley,” which represents an eastward extension of the traditional "Tornado Alley" in the central Great Plains. 26 However, this could be due to the techniques used to smooth reports in space and time. 27 An eastward shift in tornado frequency was also suggested, but results were limited to two arbitrary regions, coarse spatial environmental covariates, and lacked a robust environmental tornado report proxy such as STP. 12 , 13 Using Theil-Sen slope analysis—a robust linear trend estimator which is insensitive to outliers—and Kendall’s τ test for statistical significance, we show a robust downward trend in the annual accumulation of STP across the central and southern Great Plains. Meanwhile, a robust upward trend is found in portions of the Southeast, Midwest, and Northeast (Fig. 4 ). No significant increase (decrease) in tornado environments is observed west (east) of the 95 th meridian. We believe these trends in tornado environments are significant and have not been documented with this level of detail by previous research.
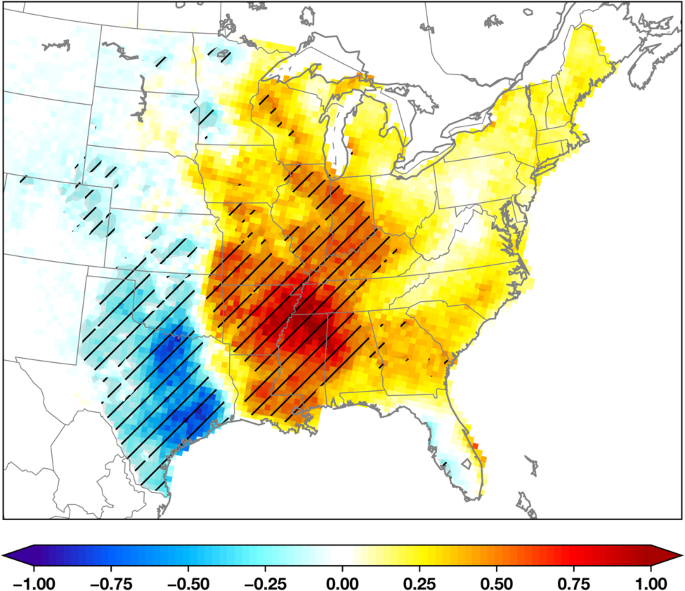
Theil-Sen slope analysis of 1979–2017 annual grid-point sum of daily max STP from NARR. p values are hatched at values ≤ 0.05 significance using Kendall’s τ statistic. Slope units are sum of daily max STP per year
Given these environmental tendencies, one would also suspect to find such evidence of trends in tornado reports given the explanatory power of the STP covariate. Indeed, significant trends in the spatial frequency of annual tornado reports are found over the analysis period (Fig. 5 ). Both tornado reports and environments indicate significant decreasing trends in frequency over portions of Texas, Oklahoma, and northeast Colorado. Agreement in the sign of the Theil-Sen slope is also noted for significant increasing trends in portions of Mississippi, Alabama, Arkansas, Missouri, Illinois, Indiana, Tennessee, and Kentucky. We are most confident about the trends in these areas, where both tornado reports and environments share the same significant sign of Theil-Sen’s slope. Confidence in the trends of tornado frequency are reduced in areas where the sign of Theil-Sen’s slope is opposite or do not share statistical significance. For example, significant increases in tornado reporting trends are found across Kansas, but environments indicate a neutral or downward trend. Additionally, tornado environments indicate a robust increasing trend in portions of Arkansas and Louisiana that are not supported by tornado reporting trends. We cannot offer a physical reason for these discrepancies at the current time, but they may be a function of non-continuous regional/seasonal efficiencies of the STP covariate (e.g., biases shown in Fig. 2 ) and biases in the tornado report database. We speculate the non-continuous regional/seasonal efficiencies of STP are a function of convective initiation and forcing for ascent. In addition, STP was designed with the intention of evaluating environments favorable for right-moving supercells in the Great Plains of the U.S. and not necessarily non-mesocyclone tornadoes. 15 , 28 However, results associated with reports herein are generally consistent with an increasing trend found in the Southeast United States due to a greater propensity for tornado outbreaks east of the Mississippi River. 29 , 30
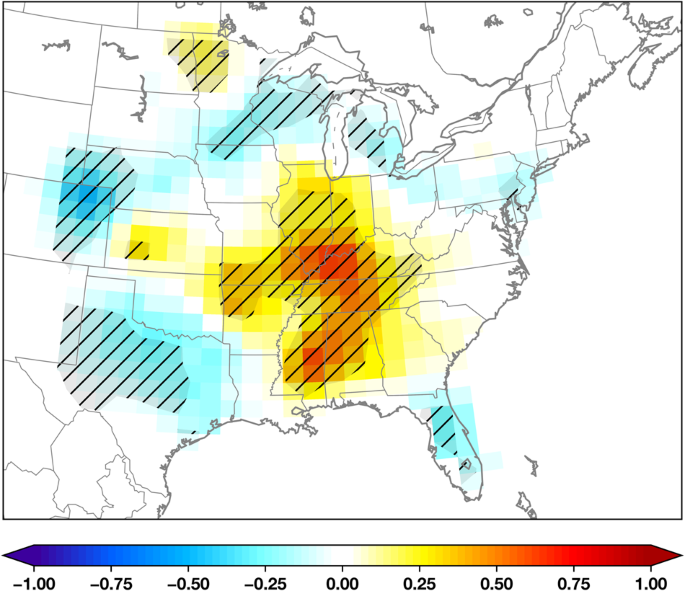
Theil-Sen slope analysis of 1979 – 2017 annual gridded tornado reports. p values are hatched at values ≤ 0.05 significance using Kendall’s τ statistic. Slope units are reports per year * 10 −1
Further examination reveals that spatial trends in tornado environment frequency are modulated by seasons (Fig. 6 ). Positive trends in tornado environment frequency are shown to progress northward through the annual cycle. Most of the decreasing trends across Texas are due to the months of March, April, and May (Fig. 6b ), and most of the Great Plains has shown a decreasing trend in tornado environments during June, July, and August (Fig. 6c ). It should be noted that our results do not indicate that maximum United States tornado environment and report frequency is no longer in the Great Plains. Rather, we demonstrate there has been a robust downward trend of tornado environments and reports in portions of the Great Plains concurrent with a simultaneous robust increasing trend in areas surrounding the Mississippi River Valley and the Midwest.
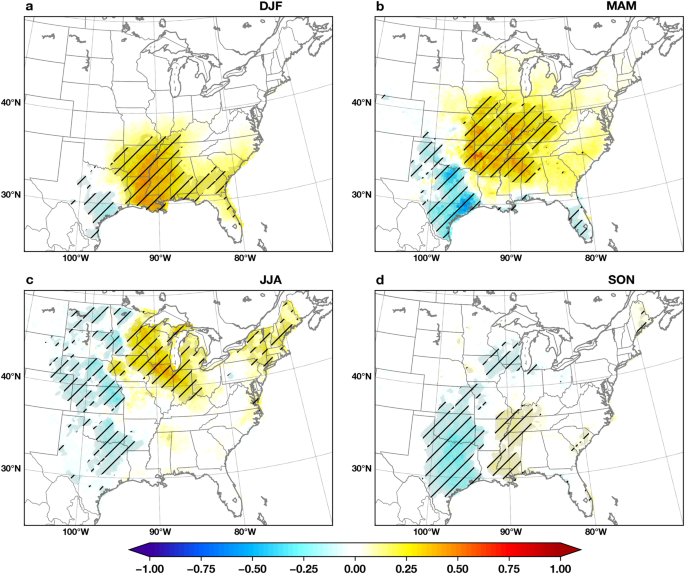
Theil-Sen slope of 1979–2017 annual grid-point sum of daily max STP for a) December, January, February; b) March, April, May; c) June, July, August; and d) September, October, November. p values are hatched at values ≤ 0.05 significance using Kendall’s τ statistic. Slope units are sum of daily max STP per year
The presented trends in spatial tornado frequency are important for understanding the tornado as a hazard, which involves examining the comingling of physical risk (i.e., climatological probability of a tornado report) and societal vulnerability (e.g., population density). Such increases in tornado frequency across the Mid-South juxtapose with an already vulnerable population to tornadoes. In fact, this geographic area of the United States already represents a maximum in the occurrence of casualties associated with tornadoes. 31 Climate downscaling and societal vulnerability studies also highlight the Mid-South as having the greatest potential for increased tornado disasters by the end of the century. 23 , 25 , 32 , 33
Economic losses associated with tornadoes will continue to increase in future years, both due to the spatial changes presented herein and the expanding footprint of the built environment. 34 It is noted that the impacts associated with projected 21 st century increases in tornado frequency are outweighed by projected growth in the human-built environment. However, the combination of an increase in risk and exposure could lead to a threefold increase in tornado disaster potential. 32 , 33 Thus, it is important to continue monitoring the presented physical trends in tornado frequency and recognize that they have consequences for increases in tornado disaster potential.
At this point, it is unclear whether the observed trends in tornado environment and report frequency are due to natural variability or being altered by anthropogenic forcing on the climate system. Global and regional climate model studies indicate a general increase in severe weather frequency (especially in boreal spring) and variability by the end of the 21st century in portions of the Midwest and Southeast, suggesting that the trends herein may indeed be due to anthropogenic forcing given the accumulating literature examining tornado/severe weather frequency and variability. 3 This eastward shift is also consistent with recent work suggesting an eastward shift in the “effective 100th meridian” as the century progresses. 35 It is difficult to isolate these natural vs. anthropogenic factors, but perhaps future attribution studies will be able to describe such causal mechanisms.
Tornado data were retrieved from the Storm Prediction Center’s online database (described online at http://www.spc.noaa.gov/wcm/#data ] for the period 1979–2017. All tornadoes were used in this analysis. Data from the North American Regional Reanalysis (NARR; 36 ) was used to calculate the STP (a unitless derived environmental variable) covariate. 3-hourly data were downloaded from the NCAR/UCAR research data archive portal ( https://rda.ucar.edu/datasets/ds608.0/ ) for the period 1979–2017.
STP was calculated following a fixed-layer 15 approach:
using surface-based convective available potential energy (SBCAPE), surface-based lifting condensation level (SBLCL), 0–1 km storm relative helicity (SRH01), and 0–6 km bulk wind difference (BWD06). Since NARR data use an isobaric vertical coordinate system, data were vertically interpolated from NARR isobaric levels to AGL height coordinates for AGL calculations (e.g., 0–6 km). In addition, STP was masked in the presence of convective inhibition < −50 J kg −1 in an attempt to examine environments most favorable for tornadoes. STP was computed on the native NARR 32-km lambert conformal grid. An accumulated STP metric was derived as a covariate for comparison to the annual tornado report counts by finding the diurnal (1200–1200 UTC) max STP value from NARR 3-hourly data and summing all CONUS land point daily maximums over the year to create annual accumulation values. 1200–1200 UTC was chosen for the daily cycle to capture diurnally driven supercell and tornado climatology. 37 Annual diurnal max accumulations were used to explain annual tornado report variability. For monthly regressions, a standardized anomaly was calculated for both monthly tornado counts and monthly accumulation of diurnal maximum STP. Regression slopes of these standardized anomaly relationships were examined to demonstrate monthly explanatory capability, variability, and bias.
For spatial trend analysis of the STP covariate, a Theil-Sen estimator was calculated at each native NARR grid point on the interannual accumulation values. Theil-Sen slope analysis was chosen due its efficient computation and insensitivity to outliers. 38 Significance of the Theil-Sen slope was assessed using Kendall’s τ statistic and a p value at the 0.05 significance level. Since tornado reports are point objects in space and time—and not gridded—reports were binned in space on a 1° latitude by 1° longitude grid using the same lambert conformal projection as NARR data and smoothed in space using a 1-σ Gaussian kernel. Theil-Sen slope analysis and Kendall’s τ statistic were then calculated on interannual gridded tornado reports using the same methodology for accumulated STP. The authors note that due to the number of statistical tests, familywise error rate, and inherent autocorrelation in time and space of climatological data, such analyses are potentially impacted by the multiple testing problem. 38
Data availability
Tornado reports are available from the storm events database at http://www.spc.noaa.gov/wcm/#data . NARR data were downloaded from the Research Data Archive (RDA) at the National Center for Atmospheric Research (NCAR), Computational and Information Systems Laboratory (CISL) at http://rda.ucar.edu/datasets/ds608.0/ .
Verbout, S. M., Brooks, H. E., Leslie, L. M. & Schultz, D. M. Evolution of the US tornado database: 1954–2003. Weather Forecast. 21 , 86–93 (2006).
Article Google Scholar
Kunkel, K. E. et al. Monitoring and understanding trends in extreme storms: state of knowledge. Bull. Am. Meteorol. Soc. 94 , 499–514 (2013).
Tippett, M. K., Allen, J. T., Gensini, V. A. & Brooks, H. E. Climate and hazardous convective weather. Curr. Change Rep. 1 , 60–73 (2015).
Long, J. A., Stoy, P. C. & Gerken, T. Tornado seasonality in the southeastern United States. Weather Clim. Extrem. 20 , 81–91 (2018).
Brooks, H. E., Carbin, G. W. & Marsh, P. T. Increased variability of tornado occurrence in the United States. Science 346 , 349–352 (2014).
Tippett, M. K. Changing volatility of US annual tornado reports. Geophys. Res. Lett. 41 , 6956–6961 (2014).
Farney, T. J. & Dixon, P. G. Variability of tornado climatology across the continental United States. Int. J. Climatol. 35 , 2993–3006 (2015).
Guo, L., Wang, K. & Bluestein, H. B. Variability of tornado occurrence over the continental United States since 1950. J. Geophys. Res. Atmos. 121 , 6943–6953 (2016).
Tippett, M. K., Lepore, C. & Cohen, J. E. More tornadoes in the most extreme US tornado outbreaks. Science 354 , 1419–1423 (2016).
Long, J. A. & Stoy, P. C. Peak tornado activity is occurring earlier in the heart of “Tornado Alley”. Geophys. Res. Lett. 41 , 6259–6264 (2014).
Lu, M., Tippett, M. & Lall, U. Changes in the seasonality of tornado and favorable genesis conditions in the central United States. Geophys. Res. Lett. 42 , 4224–4231 (2015).
Agee, E., Larson, J., Childs, S. & Marmo, A. Spatial redistribution of US Tornado activity between 1954 and 2013. J. Appl. Meteorol. Climatol. 55 , 1681–1697 (2016).
Moore, T. W. Annual and seasonal tornado trends in the contiguous United States and its regions. Int. J. Climatol. 38 , 1582–1594 (2018).
Johns, R. H. & Doswell, C. A. III Severe local storms forecasting. Weather Forecast. 7 , 588–612 (1992).
Thompson, R. L., Edwards, R., Hart, J. A., Elmore, K. L. & Markowski, P. Close proximity soundings within supercell environments obtained from the Rapid Update Cycle. Weather Forecast. 18 , 1243–1261 (2003).
Tippett, M. K., Sobel, A. H., Camargo, S. J. & Allen, J. T. An empirical relation between US tornado activity and monthly environmental parameters. J. Clim. 27 , 2983–2999 (2014).
Rasmussen, E. N. & Blanchard, D. O. A baseline climatology of sounding-derived supercell and tornado forecast parameters. Weather Forecast. 13 , 1148–1164 (1998).
Rasmussen, E. N. Refined supercell and tornado forecast parameters. Weather Forecast. 18 , 530–535 (2003).
Gensini, V. A. & Ashley, W. S. Climatology of potentially severe convective environments from the North American regional reanalysis. E J. Severe Storms Meteorol. 6 , 1–40 (2011).
Google Scholar
Trapp, R. J., Robinson, E. D., Baldwin, M. E., Diffenbaugh, N. S. & Schwedler, B. R. Regional climate of hazardous convective weather through high-resolution dynamical downscaling. Clim. Dyn. 37 , 677–688 (2011).
Mahoney, K., Alexander, M. A., Thompson, G., Barsugli, J. J. & Scott, J. D. Changes in hail and flood risk in high-resolution simulations over Colorado’s mountains. Nat. Clim. Change 125 , 125–131 (2012).
Gensini, V. A. & Mote, T. L. Estimations of hazardous convective weather in the United States using dynamical downscaling. J. Clim. 27 , 6581–6589 (2014).
Gensini, V. A. & Mote, T. L. Downscaled estimates of late 21st century severe weather from CCSM3. Clim. Change 129 , 307–321 (2015).
Robinson, E. D., Trapp, R. J. & Baldwin, M. E. The geospatial and temporal distributions of severe thunderstorms from high-resolution dynamical downscaling. J. Appl. Meteorol. Climatol. 52 , 2147–2161 (2013).
Hoogewind, K. A., Baldwin, M. E. & Trapp, R. J. The Impact of climate change on hazardous convective weather in the United States: insight from high-resolution dynamical downscaling. J. Clim. 30 , 10081–10100 (2017).
Dixon, P. G., Mercer, A. E., Choi, J. & Allen, J. S. Tornado risk analysis: Is Dixie alley an extension of tornado alley? Bull. Am. Meteorol. Soc. 92 , 433–441 (2011).
Marsh, P. T. & Brooks, H. E. Comments on “Tornado risk analysis: is Dixie Alley an extension of Tornado Alley?”. Bull. Am. Meteorol. Soc. 93 , 405–407 (2012).
R. Davies-Jones, R. J., Trapp, H. B. Bluestein, tornadoes, and tornadic storms. in Severe Convective Storms (Charles A. Doswell III eds.) 167–221 (American Meteorological Society, Boston, MA, 2001).
Childs, S. J., Schumacher, R. S. & Allen, J. T. Cold-season tornadoes: climatological and meteorological insights. Weather Forecast. 33 , 671–691 (2018).
Moore, T. W. On the temporal and spatial characteristics of tornado days in the United States. Atmos. Res. 184 , 56–65 (2017).
Ashley, W. S. Spatial and temporal analysis of tornado fatalities in the United States: 1880–2005. Weather Forecast. 22 , 1214–1228 (2007).
Strader, S. M., Ashley, W. S., Pingel, T. J. & Krmenec, A. J. Projected 21st century changes in tornado exposure, risk, and disaster potential. Clim. Change 141 , 301–313 (2017).
Strader, S. M., Ashley, W. S., Pingel, T. J. & Krmenec, A. J. Observed and projected changes in United States tornado exposure. Weather, Clim., Soc. 9 , 109–123 (2017).
Ashley, W. S. & Strader, S. M. Recipe for disaster: How the dynamic ingredients of risk and exposure are changing the tornado disaster landscape. Bull. Am. Meteorol. Soc. 97 , 767–786 (2016).
Seager, R. et al. Whither the 100th meridian? The once and future physical and human geography of America’s Arid–Humid Divide. Part II: the meridian moves east. Earth Interact. 22 , 1–24 (2018).
Mesinger, F. et al. North American regional reanalysis. Bull. Am. Meteorol. Soc. 87 , 343–360 (2006).
Kelly, D., Schaefer, J., McNulty, R., Doswell, C. III & Abbey, R. Jr An augmented tornado climatology. Mon. Weather Rev. 106 , 1172–1183 (1978).
Wilcox, R. R. Fundamentals of Modern Statistical Methods: Substantially Improving Power And Accuracy . (Springer Science & Business Media, New York. 2010).
Download references
Acknowledgments
Funding for the publication of this manuscript was provided by the Northern Illinois University Open Access Publishing Fund.
Author information
Authors and affiliations.
Northern Illinois University, DeKalb, IL, 60115, USA
Vittorio A. Gensini
National Severe Storms Laboratory, Norman, OK, 73072, USA
Harold E. Brooks
You can also search for this author in PubMed Google Scholar
Contributions
V.A.G. designed research, conducted primary analysis, prepared figures, and wrote the manuscript. H.E.B. assisted with writing and research design.
Corresponding author
Correspondence to Vittorio A. Gensini .
Ethics declarations
Competing interests.
The authors declare no competing interests.
Additional information
Publisher's note: Springer Nature remains neutral with regard to jurisdictional claims in published maps and institutional affiliations.
Rights and permissions
Open Access This article is licensed under a Creative Commons Attribution 4.0 International License, which permits use, sharing, adaptation, distribution and reproduction in any medium or format, as long as you give appropriate credit to the original author(s) and the source, provide a link to the Creative Commons license, and indicate if changes were made. The images or other third party material in this article are included in the article’s Creative Commons license, unless indicated otherwise in a credit line to the material. If material is not included in the article’s Creative Commons license and your intended use is not permitted by statutory regulation or exceeds the permitted use, you will need to obtain permission directly from the copyright holder. To view a copy of this license, visit http://creativecommons.org/licenses/by/4.0/ .
Reprints and permissions
About this article
Cite this article.
Gensini, V.A., Brooks, H.E. Spatial trends in United States tornado frequency. npj Clim Atmos Sci 1 , 38 (2018). https://doi.org/10.1038/s41612-018-0048-2
Download citation
Received : 30 May 2018
Revised : 12 September 2018
Accepted : 24 September 2018
Published : 17 October 2018
DOI : https://doi.org/10.1038/s41612-018-0048-2
Share this article
Anyone you share the following link with will be able to read this content:
Sorry, a shareable link is not currently available for this article.
Provided by the Springer Nature SharedIt content-sharing initiative
This article is cited by
- Stephen M. Strader
- Victor A. Gensini
- Amanda N. Wagner
npj Natural Hazards (2024)
What Twisters gets right — and wrong — about tornado science
- Alexandra Witze
Nature (2024)
- Matthew Graber
- Robert J. Trapp
npj Climate and Atmospheric Science (2024)
Multi-timescale exploration of teleconnection/tornado activity relationships in the Southeastern United States
- Todd W. Moore
- Tiffany A. DeBoer
- Tyler Fricker
Theoretical and Applied Climatology (2024)
Exploring the overlap: comparing STORGIS and ONETOR data between 1950 and 1989
- Zoe Schroder
Quick links
- Explore articles by subject
- Guide to authors
- Editorial policies
Sign up for the Nature Briefing: Anthropocene newsletter — what matters in anthropocene research, free to your inbox weekly.

Information
- Author Services
Initiatives
You are accessing a machine-readable page. In order to be human-readable, please install an RSS reader.
All articles published by MDPI are made immediately available worldwide under an open access license. No special permission is required to reuse all or part of the article published by MDPI, including figures and tables. For articles published under an open access Creative Common CC BY license, any part of the article may be reused without permission provided that the original article is clearly cited. For more information, please refer to https://www.mdpi.com/openaccess .
Feature papers represent the most advanced research with significant potential for high impact in the field. A Feature Paper should be a substantial original Article that involves several techniques or approaches, provides an outlook for future research directions and describes possible research applications.
Feature papers are submitted upon individual invitation or recommendation by the scientific editors and must receive positive feedback from the reviewers.
Editor’s Choice articles are based on recommendations by the scientific editors of MDPI journals from around the world. Editors select a small number of articles recently published in the journal that they believe will be particularly interesting to readers, or important in the respective research area. The aim is to provide a snapshot of some of the most exciting work published in the various research areas of the journal.
Original Submission Date Received: .
- Active Journals
- Find a Journal
- Proceedings Series
- For Authors
- For Reviewers
- For Editors
- For Librarians
- For Publishers
- For Societies
- For Conference Organizers
- Open Access Policy
- Institutional Open Access Program
- Special Issues Guidelines
- Editorial Process
- Research and Publication Ethics
- Article Processing Charges
- Testimonials
- Preprints.org
- SciProfiles
- Encyclopedia
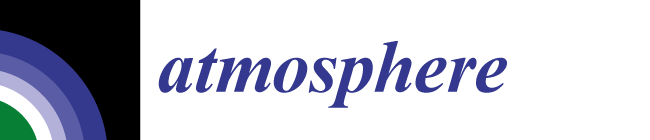
Article Menu
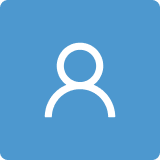
- Subscribe SciFeed
- Recommended Articles
- Google Scholar
- on Google Scholar
- Table of Contents
Find support for a specific problem in the support section of our website.
Please let us know what you think of our products and services.
Visit our dedicated information section to learn more about MDPI.
JSmol Viewer
Trend analysis of u. s. tornado activity frequency.
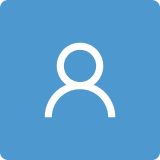
1. Introduction
2. data and methods, 2.1. observational data, 2.2. sample generation by replacement (sgr), 2.3. trend-free approach for removing ar(1) process, 2.4. non-parametric mk statistical test for trend detection, 2.5. monte carlo simulation for confirming detected trends, 3.1. trend analyses for u.s. tornadoes (e)f1–(e)f5 over the last six decades, 3.2. two-way interconnections between long-term climate trends and internal variabilities, 4. discussion and conclusions, author contributions, institutional review board statement, informed consent statement, data availability statement, acknowledgments, conflicts of interest.
- NOAA. National Weather Service. Quick Facts: Tornadoes. Available online: www.crh.noaa.gov/images/dvn/downloads/quickfacts_Tornadoes.pdf (accessed on 1 October 2018).
- NOAA. National Weather Service, Office of Climate, Water, and Weather Services. Weather Fatalities. Available online: http://www.nws.noaa.gov/om/hazstats.shtml (accessed on 1 October 2018).
- Ashley, W.S.; Strader, S.M. Recipe for disaster: How the dynamic ingredients of risk and exposure are changing the tornado. disaster landscape. Bull. Am. Meteorol. Soc. 2016 , 97 , 767–786. [ Google Scholar ] [ CrossRef ]
- IPCC. Summary for policymakers. In Climate Change 2014: Impacts, Adaptation, and Vulnerability ; Field, C.B., et al., Eds.; Cambridge University Press: Cambridge, UK, 2014; pp. 1–32. Available online: https://www.ipcc.ch/site/assets/uploads/2018/02/ar5_wgII_spm_en.pdf (accessed on 16 March 2022).
- The U.S. Climate Change Science Program and the Subcommittee on Global Change Research. In Weather and Climate Extremes in a Changing Climate ; Department of Commerce, NOAA’s National Climatic Data Center: Washington, DC, USA, 2008.
- Tippett, M.K.; Lepore, C.; Cohen, J.E. More tornadoes in the most extreme U.S. tornado outbreaks. Science 2016 , 354 , 1419–1423. [ Google Scholar ] [ CrossRef ] [ PubMed ] [ Green Version ]
- Diffenbaugh, N.S.; Trapp, R.J.; Brooks, H.E. Does global warming influence tornado activity? Eos Trans. 2008 , 89 , 553–554. [ Google Scholar ] [ CrossRef ] [ Green Version ]
- Kunkel, K.E.; Karl, T.R.; Kossin, J.; Arndt, D.; Bosart, L.; Changnon, D.; Cutter, S.L.; Doesken, N.; Emanuel, K.; Groisman, P.Y.; et al. Monitoring and understanding trends in extreme storms. Bull. Am. Meteor. 2013 , 4 , 499–514. [ Google Scholar ] [ CrossRef ]
- Verbout, S.M.; Brooks, H.E.; Leslie, L.M.; Schultz, D.M. Evolution of the U.S. tornado database: 1954-2003. Weather Forecast. 2006 , 21 , 86–93. [ Google Scholar ] [ CrossRef ]
- Mann, H.B. Nonparametric test against trend. Econometrica 1945 , 13 , 245–259. [ Google Scholar ] [ CrossRef ]
- Kendall, M.G. Rank Correlation Methods ; Charles Griffin: London, UK, 1975. [ Google Scholar ]
- Moore, T.W. On the temporal and spatial characteristics of tornado days in the United States. Atmos. Res. 2017 , 184 , 56–65. [ Google Scholar ] [ CrossRef ]
- Guo, L.; Wang, K.; Bluestein, H.B. Variability of tornado occurrence over the continental United States since 1950. J. Geophys. Res. Atmos. 2016 , 121 , 6943–6953. [ Google Scholar ] [ CrossRef ] [ Green Version ]
- Gensini, V.A.; Brooks, H.E. Spatial trends in United States tornado frequency. NPJ Clim. Atmos. Sci. 2018 , 1 , 38. [ Google Scholar ] [ CrossRef ] [ Green Version ]
- Moore, T.W. Annual and seasonal tornado trends in the contiguous United States and its regions. Int. J. Clim. 2018 , 38 , 1582–1594. [ Google Scholar ] [ CrossRef ]
- Yue, S.; Wang, C.Y. Regional streamflow trend detection with consideration of both temporal and spatial correlation. Int. J. Clim. 2002 , 22 , 933–946. [ Google Scholar ] [ CrossRef ]
- Cao, Z. Severe hail frequency over Ontario, Canada: Recent trend and variability. Geophys. Res. Lett. 2008 , 35 , L14803. [ Google Scholar ] [ CrossRef ]
- Chu, P.S.; Wang, J.B. Recent climate change in the tropical western Pacific and Indian Ocean regions as detected by outgoing longwave radiation records. J. Clim. 1997 , 10 , 636–646. [ Google Scholar ] [ CrossRef ] [ Green Version ]
- Cao, Z.; Ma, J. Summer severe rainfall frequency trend and variability over Ontario, Canada. J. Appl. Meteor. Clim. 2009 , 48 , 1955–1960. [ Google Scholar ] [ CrossRef ]
- Cao, Z.; Cai, H. Detection of tornado frequency trend over Ontario, Canada. Open Atmos. Sci. J. 2011 , 5 , 27–31. [ Google Scholar ] [ CrossRef ]
- Fuhrmann, C.M. Ranking of tornado outbreaks across the United States and their climatological characteristics. Weather Forecast. 2014 , 29 , 684–701. [ Google Scholar ] [ CrossRef ]
- Lombardi, L.; Pastore, M. Sensitivity of fit indices to fake perturbation of ordinal data: A sample by replacement approach. Multivar. Behav. Res. 2012 , 47 , 519–546. [ Google Scholar ] [ CrossRef ]
- Pastore, M.; Lombardi, L. The impact of faking on Cronbach’s Alpha for dichotomous and ordered rating scores. Qual. Quant. 2014 , 48 , 1191–1211. [ Google Scholar ] [ CrossRef ]
- Lombardi, L.; Pastore, M. sgr: A package for simulating conditional fake ordinal data. R J. 2014 , 6 , 164–177. [ Google Scholar ] [ CrossRef ]
- Anderson, C.J.; Wikle, C.K.; Zhou, Q.; Royle, J.A. Population Influences on Tornado Reports in the United States. Weather Forecast. 2007 , 22 , 571–579. [ Google Scholar ] [ CrossRef ] [ Green Version ]
- Elsner, J.B.; Fricker, T.; Schroder, Z. Increasingly Powerful Tornadoes in the United States. Geophys. Res. Lett. 2019 , 46 , 392–398. [ Google Scholar ] [ CrossRef ] [ Green Version ]
- Agee, E.; Childs, S. Adjustments in tornado counts, F-scale intensity, and path width for assessing significant tornado destruction. J. Appl. Meteor. Clim. 2014 , 53 , 1494–1505. [ Google Scholar ] [ CrossRef ]
- Wilks, D. Statistical Methods in the Atmospheric Sciences: An Introduction ; Academic Press: Cambridge, MA, USA, 1995; p. 467. [ Google Scholar ]
- Brooks, H.E.; Carbin, G.W.; Marsh, P.T. Increased variability of tornado occurrence in the United States. Science 2014 , 346 , 349–352. [ Google Scholar ] [ CrossRef ] [ PubMed ]
- Anderson-Frey, A.K.; Richardson, Y.P.; Dean, A.R.; Thompson, R.L.; Smith, B.T. Near-Storm Environments of Outbreak and Isolated Tornadoes. Weather Forecast. 2018 , 33 , 1397–1412. [ Google Scholar ] [ CrossRef ]
- Cao, Z.; Cai, H.; Zhang, G.J. Geographic Shift and Environment Change of U.S. Tornado Activities in a Warming Climate. Atmosphere 2021 , 12 , 567. [ Google Scholar ] [ CrossRef ]
- Tippett, M.K.; Cohen, J.E. Tornado Outbreak Variability Follows Taylor’s Power Law of Fluctuation Scaling and Increases Dramatically with Severity. Nat. Commun. 2016 , 7 , 10668. [ Google Scholar ] [ CrossRef ] [ PubMed ] [ Green Version ]
- Thompson, D.W.J.; Barnes, E.A.; Deser, C.; Foust, W.E.; Phillips, A.S. Quantifying the role of internal climate variability in future climate trends. J. Clim. 2015 , 28 , 6443–6456. [ Google Scholar ] [ CrossRef ]
- Nouri, N.; Devineni, N.; Were, V.; Khanbilvardi, R. Explaining the trends and variability in the United States tornado records using climate teleconnections and shifts in observational practices. Sci. Rep. 2021 , 11 , 1741. [ Google Scholar ] [ CrossRef ]
- Elsner, J.; Michaels, L.; Scheitlin, K.; Elsner, I. The decreasing population bias in tornado reports across the Central Plains. Weather Clim. Soc. 2013 , 5 , 221–232. [ Google Scholar ] [ CrossRef ]
- Elsner, J.; Elsner, S.; Jagger, T. The increasing efficiency of tornado days in the United States. Clim. Dyn. 2015 , 45 , 651–659. [ Google Scholar ] [ CrossRef ]
- Moore, T.; DeBoer, T. A review and analysis of possible changes to the climatology of tornadoes in the United States. Prog. Phys. Geogr. Earth Environ. 2019 , 43 , 365–390. [ Google Scholar ] [ CrossRef ]
- Moore, T.; Fricker, T. Tornadoes in the USA are concentrating on fewer days, but their power dissipation is not. Theor. Appl. Climatol. 2020 , 142 , 1569–1579. [ Google Scholar ] [ CrossRef ]
Click here to enlarge figure
EF Scale | (E)F 0 | (E)F1 | (E)F2 | (E)F3 | (E)F4 | (E)F5 |
---|---|---|---|---|---|---|
V | 33.5 | 43.8 | 55.0 | 67.3 | 81.8 | 89.4 |
Speed range | 29.1–38.0 | 38.4–49.2 | 49.6–60.4 | 60.8–73.8 | 74.2–89.4 | >89.4 |
Authors | Entire or Regions of U.S. | Tornado Counts and/or Days | Trend Analysis Methods | Individual (E)F Scale or (E)F+ | Data Coverage (Years) |
---|---|---|---|---|---|
Verbout et al. (2007) | Entire | Counts | Linear Regression | (E)F0–(E)F5 (E)F1+…(E)F4+ | 1954–2003 (50 years) |
Kunkel et al. (2013) | Entire | Counts | Eye-ball Slope Line | (E)F0 (E)F1+ | 1950–2011 (62 years) |
Moore (2017) | Entire | Days | MK test with pre-whitening | (E)F1+ | 1974–2015 (42 years) |
Guo et al. (2016) | Regions | Counts | Linear Regression | (E)F1+ | 1950–2013 (64 years) |
Gensini and Brooks (2018) | Regions | Counts | Linear Regression | (E)F0+ | 1979–2017 (39 years) |
Moore (2018) | Regions | Counts | MK test with pre-whitening | (E)F1+ | 1954–2016 (63 years) |
Our work | Entire | Counts and Days | (1) Linear Regression (2) MK test with trend-free pre-whitening (3) Monte Carlo simulation | (E)F1+ (E)F1…(E)F5 | 1954–2018 (65 years) |
F Scale | (E)F1 | (E)F2 | (E)F3 | (E)F4 |
---|---|---|---|---|
t > t (=1.96) | 2.15 | 2.10 | 2.10 | 2.19 |
θ | 0.25 | 0.45 | 0.40 | 0.35 |
F Scale | (E)F1 | (E)F2 | (E)F3 | (E)F4 | (E)F5 |
---|---|---|---|---|---|
Z statistic | 3.66 | −5.83 | −4.26 | −3.06 | −1.06 |
2.58 | 2.58 | 2.58 | 2.58 | 1.65 | |
α | 0.01 | 0.01 | 0.01 | 0.01 | 0.10 |
Trend (tornado/year) | 1.99 | −2.00 | −0.45 | −0.10 | −0.01 |
F Scale | (E)F1 | (E)F2 | (E)F3 | (E)F4 |
---|---|---|---|---|
b | 3.9601 | 4.0000 | 0.2025 | 0.0100 |
RIV | 0.5770 | 0.5414 | 0.7669 | 0.8881 |
MDPI stays neutral with regard to jurisdictional claims in published maps and institutional affiliations. |
Share and Cite
Cao, Z.; Cai, H. Trend Analysis of U. S. Tornado Activity Frequency. Atmosphere 2022 , 13 , 498. https://doi.org/10.3390/atmos13030498
Cao Z, Cai H. Trend Analysis of U. S. Tornado Activity Frequency. Atmosphere . 2022; 13(3):498. https://doi.org/10.3390/atmos13030498
Cao, Zuohao, and Huaqing Cai. 2022. "Trend Analysis of U. S. Tornado Activity Frequency" Atmosphere 13, no. 3: 498. https://doi.org/10.3390/atmos13030498
Article Metrics
Article access statistics, further information, mdpi initiatives, follow mdpi.
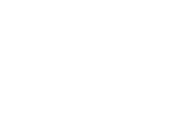
Subscribe to receive issue release notifications and newsletters from MDPI journals
Click through the PLOS taxonomy to find articles in your field.
For more information about PLOS Subject Areas, click here .
Loading metrics
Open Access
Peer-reviewed
Research Article
Tornado Intensity Estimated from Damage Path Dimensions
* E-mail: [email protected]
Affiliation Department of Geography, Florida State University, Tallahassee, FL, United States of America
Affiliation Digital Worlds Institute, University of Florida, Gainesville, FL, United States of America
- James B. Elsner,
- Thomas H. Jagger,
- Ian J. Elsner
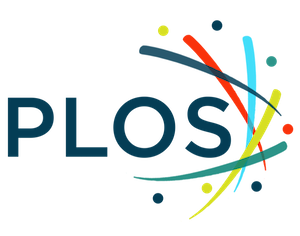
- Published: September 17, 2014
- https://doi.org/10.1371/journal.pone.0107571
- Reader Comments
The Newcastle/Moore and El Reno tornadoes of May 2013 are recent reminders of the destructive power of tornadoes. A direct estimate of a tornado's power is difficult and dangerous to get. An indirect estimate on a categorical scale is available from a post-storm survery of the damage. Wind speed bounds are attached to the scale, but the scale is not adequate for analyzing trends in tornado intensity separate from trends in tornado frequency. Here tornado intensity on a continuum is estimated from damage path length and width, which are measured on continuous scales and correlated to the EF rating. The wind speeds on the EF scale are treated as interval censored data and regressed onto the path dimensions and fatalities. The regression model indicates a 25% increase in expected intensity over a threshold intensity of 29 m s −1 for a 100 km increase in path length and a 17% increase in expected intensity for a one km increase in path width. The model shows a 43% increase in the expected intensity when fatalities are observed controlling for path dimensions. The estimated wind speeds correlate at a level of .77 (.34, .93) [95% confidence interval] with a small sample of wind speeds estimated independently from a doppler radar calibration. The estimated wind speeds allow analyses to be done on the tornado database that are not possible with the categorical scale. The modeled intensities can be used in climatology and in environmental and engineering applications. Research is needed to understand the upward trends in path length and width.
Citation: Elsner JB, Jagger TH, Elsner IJ (2014) Tornado Intensity Estimated from Damage Path Dimensions. PLoS ONE 9(9): e107571. https://doi.org/10.1371/journal.pone.0107571
Editor: Duccio Rocchini, Fondazione Edmund Mach, Research and Innovation Centre, Italy
Received: October 30, 2013; Accepted: August 19, 2014; Published: September 17, 2014
Copyright: © 2014 Elsner et al. This is an open-access article distributed under the terms of the Creative Commons Attribution License , which permits unrestricted use, distribution, and reproduction in any medium, provided the original author and source are credited.
Funding: Partial support for this work came from the Department of Geography at Florida State University and from Climatek, Inc. The funder had no role in study design, data collection and analysis, decision to publish, or preparation of the manuscript.
Competing interests: The authors have declared that no competing interests exist.
Introduction
A tornado is a violently rotating column of air capable of producing catastrophic damage where it comes in contact with the ground. The United States experiences more tornadoes than any country on earth [1] . Advances in technology have improved forecasts and warnings of these events; nevertheless, the active 2011 season (with over 1700 tornadoes) took the lives of more than 550 people [2] . The devastating impacts from these events [3] make understanding and predicting them important. But the short duration and unpredictable nature of tornadoes together with extreme velocities make it difficult to obtain direct measurements of wind speeds within the vortex.
Post storm surveys of the destruction in the wake of a tornado allow engineers to rate the damage on a scale from zero to five. Historically the damage scale was related physically to the tornado wind speed [4] , [5] . Today wind speed is phenomenologically related to the observed damage [6] . The estimated wind speed is a 3 sec gust at the location of damage based on indicators of damage to structures and vegetation and includes the degree of damage taking into account differences in construction quality [7] . For instance EF1 (category one on the Enhanced Fujita scale) damage corresponds to wind speeds between 38 and 49 m s −1 and EF4 damage corresponds to wind speeds between 75 and 89 m s −1 (derived EF scale). The EF rating assigned to tornadoes in the historical record is the highest damage category found within the damage path [8] . The EF scale is consistent with the original F scale but it includes additional damage indictors and it expands on the degree of damage. The scale was formally adopted by the U.S. National Weather Service (NWS) in 2007. Studies have addressed the need for more reliable measures of tornado winds and the potential discrepancies between wind speeds estimated by radar and damage ratings [9] .
The damage rating is correlated to path length and path width [10] . Here we make use of these relationships to build a statistical model for a single ‘best’ estimate of tornado intensity. We do this by assuming a Weibull distribution for intensity and by treating the wind speed ranges on the EF scale as interval-censored data. The latter means the unobserved intensity of the tornado falls somewhere between the end points of the interval defined by each EF category. The model provides an estimate of the intensity (highest) on a continuous scale for each tornado in the database allowing climatologists to examine changes in intensity separate from changes in frequency. This is crucial for understanding the relationship between tornadoes and climate change because increasing intensity does not need to imply more strong tornadoes if frequency decreases. Moreover, intensities together with some additional assumptions can be used to estimate tornado power. This is important as results from climate models indicate that the future might include more days when high wind shear coincides with high values of convective available potential energy [11] suggesting perhaps the possibility of more powerful tornadoes.
The paper is outlined as follows. In section 2 we briefly describe the data used in this study and examine damage path relationships. Our focus is on the most recent set of years when the quality and consistency of reporting is at its highest. In section 3 we describe our statistical model for tornado intensity. We examine model fit and provide an interpretation of the coefficients. In section 4 we examine model validity and adequacy. We also consider the variation in annually-averaged tornado intensity. In section 5 we give a brief summary and provide some concluding remarks. The code to reproduce the analysis and results is available at rpubs.com/jelsner/TornadoIntensityModel.
Tornado Damage Path Relationships
The U.S. Storm Prediction Center (SPC) maintains the most up-to-date and readily available record of tornadoes in the United States compiled from NWS Storm Data publications and reviewed by the U.S. National Climate Data Center [12] . We obtain the dataset containing all reported tornadoes over the period 1950–2013 from www.spc.noaa.gov/gis/svrgis/ and subset for years beginning with 2007 when the EF scale was was adopted. According to a report by the Pacific Northwest National Laboratory for the U.S. Nuclear Regulatory Commission, the SPC database is in reasonably good condition and acceptable for use in this type of analysis [13] .
We consider all tornadoes with an EF rating for a total of 8,752 over the period 2007–2013, inclusive. Damage assessments are made using radar tracking, eyewitness accounts, media reports, and damage photos and videos. Sometimes areal and ground surveys are taken. If a tornado produces at least one fatality, numerous injuries requiring hospitalization, extensive property damage, or widespread media interest (defined as a significant event), the damage rating is determined by meteorologists and engineers after a ground and/or aerial survey. Besides the EF rating the damage assessment includes the path length and maximum path width. There were 4994 EF0, 2642 EF1, 818 EF2, 232 EF3, 57 EF4 and 9 EF5 tornado reports during the period of study and there are no significant upward or downward trends in the annual frequencies by damage rating. The average number of tornadoes per year over this period is 1250.
Damage path length and width are related to EF rating [10] as shown in Table 1 . Lengths are recorded to the nearest hundredth of a mile and widths to the nearest yard but frequently rounded to the nearest 5 or 10 yards. The width represents the widest extent of the path. We assume that the path does not include damage associated with the rear-flank downdraft. The number of violent tornadoes (EF4 and EF5) is only .7% of the total number of all tornadoes. The total range of path length and width is large even within individual EF categories. However there is a clear relationship between EF category and path length as well as between EF category and path width ( Fig. 1 ).
- PPT PowerPoint slide
- PNG larger image
- TIFF original image
https://doi.org/10.1371/journal.pone.0107571.g001
https://doi.org/10.1371/journal.pone.0107571.t001
Brooks [10] fits Weibull distributions to path length and path width by damage rating. Here we show the distributions of actual length and width since we use them as covariates in a statistical model describing their relationship with EF rating. We note that mean path length and width have increased over time so the values in Table 1 are larger than the corresponding values reported earlier in [10] based on data over the period 1950–2001. Length explains 30% of the variability in EF rating and width explains 37% of the variability. Width explains 35% of the variability in length.
Weibull regression
https://doi.org/10.1371/journal.pone.0107571.t002
Given an EF rating along with path length, path width, and whether or not there was a fatality, the model generates samples of predictive intensity ( Fig. 2 ). The plot shows histograms based on 1000 samples from 36 tornadoes since 2007. Six randomly chosen tornadoes are included from each of the six EF ratings (top row lowest to highest) with the date/time displayed in the panel heading. The histograms are bounded by the wind speeds assigned to the EF category. In some cases the histogram is flat indicating that the length and width do not provide information on tornado intensity beyond the EF rating. However there are exceptions especially for tornadoes with ratings between EF1 and EF3. Here we see cases where the distribution is positively skewed indicating that length and width suggest a lower-end intensity for the given rating.
Six randomly chosen from each of six EF ratings (top row lowest to highest). Date/time are given in the panel heading.
https://doi.org/10.1371/journal.pone.0107571.g002
Adequacy and validation
The collective predictive distribution and model residuals are used to check against model adequacy ( Fig. 3 ). The shape of the predictive distribution appears reasonable for intensity with a positive skew and a long right tail. There is a small notch in the distribution around the cutoff between EF1 and EF2 tornadoes. This is explained by relatively few high-end EF1s and relatively many low-end EF2s predicted based on path dimensions. The model residuals are approximately normally distributed with the exception being a long right tail indicating a few unusually short-lived and narrow tornadoes relative to their high assessed EF rating.
(a) Histogram of predicted tornado intensities. (b) Histogram of model residuals. (c) Quantile-normal plot of the model residuals.
https://doi.org/10.1371/journal.pone.0107571.g003
Individual predictive distributions provide a check on model validity. The vast majority of tornadoes do not have an estimated wind speed. However, in some cases it is possible to obtain a wind speed estimate from a nearby Weather Service Radar-88D measurements or from a mobile radar (Doppler on Wheels). We obtain estimates from the SPC's Storm Reports for nine tornadoes during the 2013 season and compare them to samples from our intensity model ( Fig. 4 ). The wind speeds range from a low of 56 m s −1 for the Kilpatrick, AL March 18th EF2 tornado to a high of 92 m s −1 for the New Castle/Moore OK May 20th EF5 tornado. The predictive distributions are shown as histograms. In cases where the histograms are skewed, the estimated wind speed tends to be on the corresponding side of the EF range. The exception is the Sedgwick, KS May 19th tornado. The correlation between the estimated wind speeds and the average over all predictive samples is .98 with a root-mean squared error of 5.4 m s −1 .
The location of the estimated wind speed is shown as a dot and the range of wind speeds defined by the corresponding EF category is shown as a gray horizontal bar.
https://doi.org/10.1371/journal.pone.0107571.g004
Model skill is estimated relative to a null model of choosing a random intensity within the wind speed ranges. Skill is assessed as the percentage increase in the coefficient of variation between path dimension and predicted intensities. The correlation between path length and EF rating is .552. The correlation between length and tornado intensity using our intensity model is .604 for an increase in the coefficient of variation of 20%. The correlation between length and tornado intensity from the null model is .566 for an increase in the coefficient of variation of 5%. Similar skill metrics are noted using path width.
We perform an out-of-sample test by correlating the modeled intensities from twelve tornadoes that have corresponding wind speeds estimated from radar measurements that are independent of the damage assessment. The derived radar wind speeds result from a calibration of mobile radar (Doppler on Wheels) with nearby Weather Service Radar-88D measurements. The data and method used to obtain the radar wind speeds are described in [16] . The modeled intensities correlate with the radar wind speeds at .77 (.34, .93) [95% CI]. Although this is a small sample of tornadoes the radar-estimated wind values range from a low of 38 m s −1 to a high of 91 m s −1 suggesting the potential for our estimates to be broadly applicable throughout the database.
Finally a single predictive sample for each tornado is plotted by year as a box plot ( Fig. 5 ). While the number of years is too few to ascertain a significant trend, there is an apparent increase in the upper quantiles of the annual intensity distributions resulting from the noted increases in damage path dimensions. Tornado intensity depends on updraft speeds within the parent thunderstorm and on increasing winds with height (shear) in the environment surrounding the thunderstorm [17] . Updraft speed is directly related to the available potential energy in the environment, which increases with greater low altitude heat and moisture. Upward trends in surface dew point temperature and specific humidity across the United States are coincident with upward trends in temperature especially over the tornado-prone Midwest [18] . With all else equal greater surface humidity implies greater available potential energy. Local shear can be large, even if it decreases in the mean, when waves in the upper-level flow amplify as occurs more often when warming in the Arctic outpaces warming elsewhere [19] .
https://doi.org/10.1371/journal.pone.0107571.g005
Tornadoes are capable of catastrophic damage. The Newcastle/Moore, OK tornado of May 20, 2013 and the El Reno, OK tornado just a week later are some recent examples. Direct measurements of tornado intensity are difficult and dangerous to get. Surveys rate the tornado damage on the EF scale and wind speed bounds are attached to the scale. Unfortunately the categorical scale is not adequate for analyzing tornado intensity separate from tornado frequency. Moreover, the historical database cannot directly benefit from improved surveillance technology.
Here we use path length and width which are measured on a continuous scale and which are strongly correlated to the EF category to estimate tornado intensity on a continuum. The model indicates a 25% increase in expected intensity over a threshold intensity of 29 m s −1 for a 100 km increase in path length and a 17% increase in expected intensity over the threshold for a 1 km increase in path width. The model also indicates a 43% increase in the expected intensity when fatalities are observed holding path dimensions constant. Diagnostic plots of the predictive density and residuals reveal no significant concern about model adequacy.
The modeled intensity allows analyses to be done on the tornado database not possible with the categorical scale. The predicted probabilities by EF category can be calibrated to the area affected by this level of damage and an index of tornado destructiveness would then follow naturally. The modeled intensities can be used in climatology and in environmental and engineering applications but more work needs to be done to understand the reason behind the increase in path length and width.
Acknowledgments
We thank Parks Camp from the U.S. National Weather Service Forecast Office in Tallahassee, Florida for helping us understand the protocol associated with surveys of tornado events.
Author Contributions
Conceived and designed the experiments: JBE THJ. Performed the experiments: JBE THJ. Analyzed the data: JBE THJ IJE. Contributed reagents/materials/analysis tools: JBE THJ IJE. Wrote the paper: JBE.
- 1. Grazulis TP (1990) Significant Tornadoes, 1880–1989: Discussion and analysis. Significant Tornadoes, 1880–1989. Environmental Films. URL http://books.google.com/books?id=E8hFAAAAYAAJ .
- View Article
- Google Scholar
- 4. Fujita T, Pearson AD (1973) Results of FPP classification of 1971 and 1972 tornadoes. In: Eight Conference on Severe Local Storms. pp. 142–145.
- 11. Diffenbaugh NS, Scherer M, Trapp RJ (2013) Robust increases in severe thunderstorm environments in response to greenhouse forcing. Proceedings of the National Academy of Sciences.
- 13. Ramsdell JV Jr, Rishel JP (2007) Tornado Climatology of the Contiguous United States. Technical Report NUREG/CR-4461, PNNL-15112, Pacific Northwest National Laboratory, P.O. Box 999, Richland, WA 99352.
- 19. Francis JA, Vavrus SJ (2012) Evidence linking arctic amplification to extreme weather in midlatitudes. Geophysical Research Letters: L06801.

An official website of the United States government
The .gov means it’s official. Federal government websites often end in .gov or .mil. Before sharing sensitive information, make sure you’re on a federal government site.
The site is secure. The https:// ensures that you are connecting to the official website and that any information you provide is encrypted and transmitted securely.
- Publications
- Account settings
Preview improvements coming to the PMC website in October 2024. Learn More or Try it out now .
- Advanced Search
- Journal List
- Int J Environ Res Public Health


Mental Health Impacts of Tornadoes: A Systematic Review
Associated data.
These data used to support the findings of this study are included within the article.
Tornadoes are one of the most prevalent natural hazards in the United States, yet they have been underrepresented in the disaster mental health comprehensive literature. In the current study, we systematically reviewed available scientific evidence within published research journals on tornadoes and mental health from 1994 to 2021. The electronic search strategy identified 384 potentially relevant articles. Of the 384 articles, 29 articles met the inclusion criteria, representing 27,534 participants. Four broad areas were identified: (i) Mental health impacts of tornadoes; (ii) Risk factors; (iii) Protective factors; and (iv) Mental health interventions. Overall, results showed adverse mental health symptoms (e.g., post-traumatic stress disorder, depression, anxiety) in both adult and pediatric populations. A number of risk factors were found to contribute to negative mental health, including demographics, tornado exposure, post-tornado stressors, and prior exposure to trauma. Protective factors found to contribute to positive outcomes included having access to physical, social, and psychological resources. Together, these findings can serve as an important resource for future mental health services in communities experiencing tornadoes.
1. Introduction
In the United States, there are more than 1200 tornadoes every year, contributing to more than 15,000 tornado-related fatalities since 1900 [ 1 , 2 ]. The average number of tornadoes has been increasing since 1954, and the likelihood of extreme tornadoes is also increasing [ 3 ], which could exacerbate the severity of the losses and negative effects of tornadoes in the future. While being directly exposed to a tornado can result in death, injuries, and physical damage to buildings and property, it can also have long-term effects on people’s mental health [ 4 ]. Emotional distress, anxiety, depression, post-traumatic stress, sleep disorder, and suicidal ideation have been reported as mental health problems experienced by victims after severe weather events [ 5 , 6 , 7 ]. These psychological symptoms can occur in the immediate aftermath of exposure and can persist over months to years [ 8 ]. In addition, people living with adverse mental health symptoms in post-extreme weather events reported a lower quality of life and functional impairments in social, vocational, and physical areas [ 9 ].
Compared to other types of frequent natural hazards (e.g., hurricanes, floods), comprehensive studies on the mental health impacts of tornadoes are lacking. For example, multiple systematic reviews have been conducted on the mental health impacts of hurricanes [ 10 , 11 ] and floods [ 12 , 13 ]. To the best of our knowledge, there have been no systematic reviews conducted and published on the mental health impacts of tornadoes. Therefore, we aimed to systematically identify and synthesize the available scientific literature on the mental health impacts of tornadoes. These findings can be used to (1) understand the mental health impacts of tornadoes, (2) identify possible risk and protective factors associated with psychological impacts, and (3) identify gaps in the literature to inform future research.
2. Materials and Methods
2.1. literature search.
Using recommendations from the PRISMA group, we conducted a systematic review to identify and summarize findings of the peer-reviewed literature on tornadoes and mental health. We carried out literature searches for this topic through APA PsycInfo ® , PUBMED, SCOPUS, and Web of Science. The search was restricted from January 1994 to December 2021 because after the Southeastern United States Palm Sunday Tornado Outbreak of 27 March 1994, there has been an increasing focus on empirical evidence-based research. We used free text, and words were restricted to title and abstract. The word “tornado” and “intervention” had an asterisk (*) added as a wildcard in to pick up plurals. The search strategy was:
Tornado* AND Psychological (Title/Abstract) OR “Mental health” (Title/Abstract) OR PTSD (Title/Abstract) OR “Posttraumatic disorder” (Title/Abstract) OR “Post-traumatic stress disorder” (Title/Abstract) OR PTSS (Title/Abstract) OR “Post-traumatic stress syndrome” OR Anxiety (Title/Abstract) OR Depression (Title/Abstract) OR “Disaster mental health” (Title/Abstract) OR “Intervention* AND Mental (Title/Abstract)”
2.2. Eligibility Criteria
All articles focused on the effects of tornadoes on mental health were included. Studies that explored the mental health impacts of tornadoes along with other disasters (e.g., tornadoes and hurricanes, tornadoes, and floods) were excluded. Articles that focused solely on the effects of tornadoes on the environment were also excluded. We included all articles dealing with: (i) mental health impact during and after tornado exposure; (ii) risk and protective factors that affect mental health during and after tornado exposure; (iii) evaluation tools and interventions to mitigate the impact of tornadoes on mental health. The following study designs were included in the systematic mapping (i) quantitative methods: survey, secondary data analysis, and data analysis after interviews; (ii) qualitative studies: individual interview; (iii) mixed methods: focus group interview (FGI) and survey, individual interview and survey. A protocol paper, case reports, letters, and editorials were excluded. We included only articles written in English.
2.3. Study Selection
Studies were screened for inclusion in two stages. The first author went over the title and published abstracts and made a preliminary list of articles in phase one. The authors assessed all articles published after 1994 and chose those that they thought fulfilled the inclusion criteria for full-text reading. Articles that were published before 1994 were excluded for two reasons: (i) to include the most recent literature; and (ii) to avoid possible inconsistencies in the description of mental disorders, as it was in 1994 that the 4th version of the Diagnostic and Statistical Manual of Mental Disorders was published [ 10 ]. When there was a disagreement, the researchers discussed their different viewpoints and came to an agreement. The authors assessed the entire publications that matched the eligibility criteria in the second phase.
2.4. Data Extraction
The first author extracted essential features from journals that met the inclusion criterion, such as methods, characteristics, and results. The co-author reviewed the essential features, study design, demographics, and a brief overview of the findings. Based on the key features and a summary of studies, the authors identified four broad categories of the topic.
2.5. Assessment of the Risk of Bias
To assess for bias in the studies, the Hoy Risk of Bias Tool (RoBT) [ 14 ] was used. The RoBT consists of ten items evaluating external (four items) and internal (six items) validity. In this review, we adopted eight items of the tool. Two of the six questions for evaluating internal validity were not adopted because they were not applicable to evaluating the articles reviewed in this paper. Studies were classified as having a low risk of study bias when six or seven of the eight items were answered as “yes (low risk),” and moderate risk of bias when three to five of the questions were answered as “yes (low risk),” and a high risk of bias when zero to two questions were answered as “yes (low risk)”. Seventeen studies had a rating of low bias, eleven studies had a rating of moderate bias, and one had a high-risk rating. A result of the assessment of the risk of bias is available in Table S1 Supporting Information.
3.1. Literature Search and Study Selection
The electronic search strategy identified 384 potentially relevant articles. After removing duplicates and irrelevant, or those that were published before January 1994, 61 were reviewed by reading the title or abstract. We assessed 35 full-text journals for eligibility, and 29 were included for further review ( Figure 1 ). A summary of the journals selected is available in Table S2 Supporting Information.

Flow diagram.
3.2. Characteristics of the Studies Selected
We found that 23 of the articles (79.3%) used quantitative methods. Of these, 21 were surveys. Three (10.4%) were qualitative studies (individual interviews) dealing with the subjective experiences of tornado exposure. Three (10.4%) articles were mixed methods of survey and focus group interviews or individual interviews. Regarding the demographics of the articles, 22 articles (75.9%) focused on children, adolescents, and their caregivers. Five (17.2%) focused on adults, and three (10.4%) focused on the general population. However, we were not able to find research that focuses on the elderly population. Twenty-one articles (72.4%) were focused on the Missouri and Alabama tornado outbreak in 2011, and five (17.2%) were focused on tornadoes in Oklahoma. One was focused on the Minnesota tornado in 1998. One study that focused on the tornado in Jiangsu, China, in 2016, was found. (See Table 1 ).
Summary of the characteristics of included references.
Types of Study | No. in References | N | % | Total No. of Participants | |
---|---|---|---|---|---|
Quantitative methods | Survey | [ , , , , , , , , , , , , , , , , , , , , ] | 21 | 72.4 | 23,260 |
Secondary data analysis | [ ] | 1 | 3.4 | 2000 | |
Data analytic after interviews | [ ] | 1 | 3.4 | 118 | |
Qualitative methods | Individual interview | [ , , ] | 3 | 10.4 | 435 |
Mixed methods | FGI and survey | [ ] | 1 | 3.4 | 1561 |
Individual interview and Survey | [ , ] | 2 | 6.9 | 160 | |
Characteristics of the Sample | |||||
Age | Children | [ , , , ] | 4 | 13.8 | 614 |
Children and parents (or caregivers) | [ , , ] | 3 | 10.4 | 2396 | |
Adolescents | [ , , , , , , ] | 7 | 24.1 | 6965 | |
Adolescents and parents (or caregivers) | [ , , , , , , ] | 7 | 24.1 | 11,515 | |
Adults | [ , , , , ] | 5 | 17.2 | 5782 | |
General | [ , , ] | 3 | 10.4 | 262 | |
Location, year, and EF scale of Tornadoes | |||||
The U.S. | Missouri and Alabama (2011) | [ , , , , , , , , , , , , , , , , , , , ] | 20 | 69.2 | 22,922 |
Oklahoma (2001) | [ ] | 1 | 3.4 | 198 | |
Oklahoma (2006) | [ , ] | 2 | 6.9 | 204 | |
Oklahoma (2013) | [ , ] | 2 | 6.9 | 181 | |
Middle Tennessee (2020) | [ ] | 1 | 3.4 | 221 | |
Minnesota (1998) | [ ] | 1 | 3.4 | 2000 | |
Non-specific | [ ] | 1 | 3.4 | 1561 | |
Outside of the U.S. | China (2016) | [ ] | 1 | 3.4 | 247 |
EF scale | EF5 | [ , , , , , , , , , , , , , , , , , , , ] | 20 | 69.2 | 24,488 |
EF4 | [ , , , , , ] | 6 | 20.7 | 1235 | |
EF3 | [ ] | 1 | 3.4 | 198 | |
Not specified | [ , ] | 2 | 6.9 | 1613 |
3.3. Main Outcomes
We analyzed 29 articles, representing 27,534 participants. Four broad areas were identified: (i) Mental health impacts of tornadoes; (ii) Risk factors; (iii) Protective factors; and (ⅳ) Mental health interventions
- Post-traumatic stress disorder (PTSD): We found in multiple studies that residents exposed to tornadoes reported PTSD or PTSD-related symptoms. Degree of tornado exposure, gender, age, emotional support, and barriers to a tornado warning were factors related to post-tornado PTSD [ 4 , 15 , 17 , 19 , 21 , 25 , 26 , 32 , 35 , 38 , 39 ]. PTSD, or symptoms associated with PTSD, was also found to be related to other mental and behavioral health needs such as depression [ 20 , 21 , 25 ], binge drinking [ 16 ], or substance abuse [ 15 ]. Studies found women were more likely to report more PTSD symptoms than men after a tornado [ 4 , 21 , 35 , 39 ], and one study found men were more likely to report depression symptoms following the Joplin 2011 tornado [ 25 ]. The questionnaires to measure PTSD were a modified version of the SF-12, The National Survey of Adolescents Replication PTSD Modules, OSU PTSD Scale-CF, the Post-traumatic Stress Disorder Checklist for Civilians, the Trauma Screening Questionnaire, Frederick Reaction Index, and the Child PTSD Symptom Scale and The UCLA PTSD index.
- Depression: We found that depression was also a common mental health consequence experienced months after the tornado [ 20 , 21 , 38 ], but also 2.5 years later [ 25 ]. Adolescents who reported two or more depressive symptoms had 3.5-fold odds of increased risk of PTSD [ 21 ]. In addition, low education, low social support, and tornado exposure increased the likelihood of a diagnosis of depression [ 20 , 21 , 25 ]. Questionnaires used to assess depression included a revised version of SF-12, the Patient Health Questionnaire-2 Depression Subscale, the Patient Health Questionnaire-8, The National Survey of Adolescents-Replication PTSD and MDD Modules, and the NSA-Depression module.
- Anxiety: Several studies reported an elevated prevalence of generalized anxiety disorder following tornadoes. In six out of 29 studies [ 22 , 28 , 38 , 39 , 40 , 42 ], the level of anxiety was measured using the modified Differential Sentiment Scale, the OSU PTSD Scale, or was measured through an interview. Anxiety experienced by residents after the tornado was also thought to be a contributing factor to PTSD symptoms [ 22 , 39 ]. For children, the degree of exposure to the tornado was found to be associated with anxiety level [ 28 ]. Measures to assess anxiety in children included the Generalized Anxiety Disorder-7.
- Alcohol and substance abuse: Studies examining increased alcohol consumption in adolescents after tornadoes were found to be significantly influenced by previous trauma history and current levels of tornado-related PTSD [ 16 , 31 ]. We found conflicting results on whether alcohol abuse was predicted by age and gender. One study reported higher levels of alcohol consumption among men and older adolescents [ 16 ]. However, in another study, gender was not a significant predictor of binge drinking, nor did it act as a risk factor for increased alcohol use after a tornado [ 31 ]. Rutgers Alcohol Problem Index and Quantity-Frequency Index of Alcohol Consumption questionnaires were used to identify problems experienced as a result of drinking. Substance use disorder (SUD) was found to be associated with post-traumatic stress disorder and major depressive episodes following exposure to tornadoes. Girls were significantly more likely than boys to meet the diagnosis criteria of comorbidity for “PTSD and major depressive episode”, and “major depressive episode and substance use disorder”. Adolescent SUD was assessed using the CRAFFT screening test [ 15 ].
- Suicidal ideation: About 5% of 2,000 adolescents who experienced the Joplin and Tuscaloosa tornadoes in the spring of 2011 reported suicidal thoughts [ 33 ]. Intimate partner violence (IPV) exposure was also found to be significantly related to post-tornado suicidal ideation, even after accounting for current mental health symptoms (i.e., PTSD and depression). Notably, prior disaster exposure and demographic characteristics were not significantly associated with suicidal ideation, suggesting that certain factors of IPV may predict suicide risk.
- Children’s emotions and distress: Avoidance, re-experiencing, interpersonal alienation, interference with daily functioning, physical symptoms/anxiety, and foreshortened future were found to be elements of mental health impacts of tornadoes upon children [ 37 ]. Studies found that emotional distress (i.e., fear) and the amount of damage to schools were also associated with overall psychological effects on children. In children, a combination of attributions, particularly meaning-seeking and perceived tornado exposure, were overall predictors of long-term distress after a tornado [ 27 ]. In addition, children’s emotional processing was found to impact children’s meaning-making efforts and have implications for their adjustment after a tornado [ 23 , 41 ]. Finally, maternal support was found to be important in the relationship between children’s use of both positive and negative emotional language and child tornado-related post-traumatic stress syndrome (PTSS). Maternal support included asking their children questions, making follow-up statements, repeating back content by paraphrasing, and providing possible solutions to problems [ 36 ].
- Gender and age: Multiple studies found females with low levels of education and lower household income were more vulnerable to adverse mental health after tornado events [ 25 , 38 , 39 ]. Children of parents who suffer from adverse mental health conditions (e.g., depression) after a disaster often experience similar difficulties [ 24 , 29 ]. In examining the relationship between age and PTSD in children and adolescents, we found two conflicting results. In one study, young children between the ages of 4 and 10 were more likely to experience PTSD after a tornado [ 24 ], while another study found that older adolescents were more likely to report symptoms of PTSD [ 29 ]. For boys, the effect of tornado exposure on PTSD and depression increased as social support decreased. However, for girls, regardless of the level of tornado exposure, social support was associated with PTSD and depression [ 29 ].
- Race and ethnicity: Two articles addressed specific challenges that the African-American and Latinx populations faced in the context of tornadoes [ 4 , 39 ]. Black and Latinx residents who reported difficulties receiving tornado warning alerts experienced more tornado exposure and adverse mental health impacts, and the intersections of ethnicity, language difficulties, citizenship, pre-mental health symptoms, and social class were also found to contribute to additional stressors [ 4 ].
- Economic factors: Having lower income, renting, having debt, not having insurance, and loss of material resources were the significant risk factors for post-tornado adverse mental health symptoms [ 19 , 24 , 25 , 29 , 39 ]. However, there was one contradictory finding that, during long-term recovery from the Oklahoma Moore Tornado in 2013, loss of personal characteristics such as self-esteem or confidence were all statistically significantly associated with adverse mental health, whereas material loss did not correlate with mental health outcomes [ 19 ].
- Tornado exposure: Multiple studies have identified that tornado exposure (e.g., injury, losing a loved one, property damage) is a strong factor that is linked to negative mental health for children, youth, and adults [ 15 , 17 , 19 , 22 , 24 , 25 , 27 , 28 , 29 , 31 ]. Families who experienced greater severity of tornado exposure had youth who reported more PTSD and caregivers who reported more distress [ 15 , 17 ]. Greater severity of tornado exposure was also associated with alcohol use by adolescents [ 16 , 31 ]. However, two studies found that tornado exposure was unrelated to child-reported emotions and mental health symptoms after a tornado [ 23 , 41 ]. Tornado Exposure Questionnaire, The Tornado-Related Traumatic Experiences Questionnaire, and Natural Disaster Experiences Inventory were used to measure the severity of tornado exposure.
- Pre-tornado trauma experience: Past trauma exposure (e.g., prior exposure to natural hazards, prior exposure to accidents) and IPV were risk factors for post-tornado mental health symptoms [ 30 ]. Among adolescents, prior exposure to traumatic events was the most consistent predictor of each post-tornado comorbidity (comorbid post-traumatic stress disorder, major depressive episode, and substance use disorder) profile [ 15 ]. Females and adolescents with an IPV history in addition to a natural hazard experience, had increased PTSD symptoms [ 30 , 35 ].
- Lack of mental health services: Finally, one study examined accessing mental health services in the 2.5 years following the 2011 Joplin, Missouri tornado [ 25 ]. 2.5 years after the tornado, the majority of respondents reported they had not accessed mental health support, and had little or no contact with a counselor or other mental health professional (83.4%) or religious leader (85.9%).
- Coping response factors: Coping responses found to have a positive impact on personal recovery and mental health included dispositional optimism, self-efficacy, and hope. Such coping responses were found to moderate the association between the severity of home damage and personal recovery, as well as the relationship between home damage and PTSD [ 18 ]. Another study found that individuals’ religiosity and emotional coping in response to tornadoes predicted taking protective action during tornadoes. Related to emotions, anxiety and fear were found to contribute to protective decision-making (e.g., sheltering in place or collecting supplies), which was then related to positive mental health outcomes [ 40 ].
- Social support: Multiple studies found that social resources were positively associated with higher levels of resilience and negatively related to adverse mental health outcomes following tornadoes [ 16 , 24 , 32 , 37 ]. One study that focused on disaster interpersonal communication and post-traumatic stress following the 2011 Joplin, Missouri tornado found that post-traumatic stress symptoms (PTS) were associated with more frequent communication with family, friends, and neighbors about the tornado [ 24 ]. Another study found that environments with fewer social resources and support influenced the risk of alcohol consumption and alcohol use disorders of residents [ 16 ]. Finally, perceived social support was found to reduce PTSD symptoms and increase Post-traumatic Growth (PTG) among participants affected by the tornado in Yancheng, Jiangsu, China [ 32 ].
- Journey of Hope: “Journey of Hope” is a program designed for young people exposed to disasters who do not meet the diagnostic criteria for formal mental health symptoms but experience emotional distress. This intervention uses a school-based psychosocial curriculum that includes eight sessions aimed at improving the emotional management of children and adolescents by improving protective parameters such as social support, coping, and psychological education. We found one study that explains 110 participants ranging from 11 and 15 years old who experienced Moore, Oklahoma tornado in 2013 improved their ability to prosocial behaviors after completing the program. According to the study, a statistically significant increase in the Strengths and Difficulties Questionnaire subscale for prosocial behaviors was found following the completion of the intervention [ 42 ].
- Bounce Back Now: “Bounce Back Now (BBN)” is a web-based intervention and consists of five standalone modules that intervene with PTSD, depression (mood), smoking, alcohol use, and parenting for adolescents at risk for post-disaster mental health problems. We found a population-based randomized controlled trial of BBN. Two thousand adolescents and parents who were affected by the tornado outbreak in Joplin and several areas in Alabama enrolled in the intervention, and nearly half of the disaster-affected families accessed the intervention. Youth and their parents were randomly assigned to (a) a web intervention for disaster-affected youth, (b) a web intervention for disaster-affected families (youth and parents), and (c) an evaluation-only web comparative experiment. Researchers found fewer PTSD and depressive symptoms for adolescents in the experimental versus control conditions at a 12-month follow-up. Results revealed the feasibility and initial efficacy of BBN as a scalable post-disaster mental health intervention for adolescents [ 34 ].
4. Discussion
To the best of our knowledge, this is the first systematic review that focuses on reviewing and synthesizing the literature on the mental health impact of tornadoes. After reviewing a total of 29 papers representing 27,534 participants, we identified four broad areas: mental health impacts of tornadoes, risk factors, and protective factors and mental health interventions. In terms of mental health impacts, we found tornadoes can have negative effects on mental health, including PTSD, anxiety, depression, suicidal thoughts, and alcohol and drug abuse in children, adolescents, and adults. Individual factors such as gender, age, ethnicity, economic status, exposure to tornadoes, pre-tornado traumatic experiences, and the social context that prevents post-disaster victims from accessing mental health services were all found to be risk factors for post-tornado mental health problems. We found that women, children, and adolescents have a relatively higher incidence of PTSD and mental health symptoms than men and adults after tornadoes. These results are the same in studies of other natural hazards such as hurricanes, earthquakes, and tsunamis [ 43 , 44 ]. In addition, we found two articles that focused on Black and Latino populations. The interconnections of their race, citizenship, legal issues, language barriers, and socioeconomic status were major stressors that needed to be addressed in post-disaster services. Other risk factors found included economic factors such as having lower income, renting, having debt, not having insurance, and loss of material resources were the risk factors for post-tornado adverse mental health problems. Future research is warranted to examine various sociocultural dimensions of risk from an intersectional framework (e.g., race and ethnicity, national origin, class, physical ability, age, and living in a rural or urban environment).
In terms of protective factors, individuals’ religiosity, hope, and dispositional optimism were found to contribute to better mental health outcomes and personal recovery. In addition, material and social resources were found to serve as important protective factors. These combined findings highlight the importance of both internal (e.g., hope, optimism) and external (e.g., material resources, social support) protective factors contributing to better mental health outcomes following tornadoes. These findings can assist practitioners in identifying protective factors that guide a framework for interventions and practice models that build resilience in post-tornado settings.
We also identified two practical interventions aimed at relieving distress and emotions in children after a tornado. In the context of natural hazards, children and adolescents are characterized as one of the most vulnerable and highly dependent [ 45 , 46 ]. In addition, children and adolescents who have experienced a disaster may experience depression and post-traumatic symptoms long after the event [ 47 , 48 ]. Childhood trauma survivors present alcohol and drug dependency issues, and early onset of trauma may contrive adverse mental health symptoms such as anxiety in adulthood [ 49 ]. For these reasons, we conclude that further research into long-term recovery programs that can develop social-emotional skills in children and adolescents may be necessary, along with follow-up studies of “Bounce Back Now” and “Journey of Hope”.
Based on these findings, we identified some important research gaps. In terms of methodological aspect, of the total 29 studies, 23 studies were conducted based on a quantitative methodology. In future research, it may be required to investigate the potential for a meta-analysis to provide additional support for the findings of individual quantitative studies. We also found that the majority of studies focused on children, adolescents, and caregivers. Notably, there have been no studies on mental health after tornadoes experienced by the elderly population and people with disabilities in the past 27 years. The paradox that older people and people with disabilities are at greater risk of disasters [ 50 , 51 , 52 ] but are excluded from disaster studies strongly suggests that studies involving these populations are needed. Given the increasing likelihood of tornadoes due to climate change [ 53 ] and a growing older population worldwide [ 54 ], it is important for policymakers and practitioners to have evidence of the impact of tornadoes on the mental health of aging populations. Furthermore, we found that the LGBTQ population was also essentially invisible following tornadoes as there was no article that focused on this group. LGBTQ people are more likely to experience social isolation and to endure disrespect or harassment in places like emergency shelters in the aftermath of a disaster [ 55 ]. Therefore, future research may be needed to explore the social isolation and consequent mental health problems experienced by this group after tornadoes. In addition, experiencing more tornado exposure (e.g., being injured, losing a loved one, housing damage) was shown to be associated with risk factors for adverse mental health, including studies from above. Prior studies indicate that individuals living/sheltering in mobile/manufactured homes have a greater probability of tornado-related injuries, fatalities, and losses [ 56 , 57 ]. Future research is warranted to examine mental health outcomes among mobile/manufactured home residents exposed to tornadoes.
Finally, we found most of the studies included in this review were published in the United States and focused on EF4 or EF5 tornadoes, with many studies being related to the Missouri and Alabama tornado outbreak in 2011. Severe weather has increased over the past few decades with climate change [ 53 ], and the geographic areas with high frequencies of tornado activity are gradually shifting to the U.S. southeast region [ 58 , 59 ]. Therefore, we recommend additional studies on tornado-related mental health outcomes from populations in the southeast. We also recommend future studies examining tornado-related mental health needs in communities with low-attention tornadoes (EF 1-3) that may not warrant federal or state support but may have considerable losses and mental health needs.
In this review, we summarized the peer-reviewed literature examining mental health impacts during and after tornado events. Although the findings of this study contribute to a better understanding of the impact of tornadoes on mental health, there are certain limitations that should be addressed in future research. First, although we included the most significant mental health consequences and keywords associated with disaster mental health in our search method, such as depression, anxiety, PTSD, mental, and psychological, it is probable that some research papers that identify other outcomes were missed. Second, we only searched articles that were written in English. In future research, if we search for papers written in multiple languages, we will be able to find data on tornadoes outside the United States and their aftermath. This attempt will add diversity and depth to tornado research, which is lacking in quantitative and qualitative compared to other natural hazards research.
5. Conclusions
We systematically reviewed the available scientific evidence on the mental health effects of tornadoes within published research journals. We extracted data from 29 articles that met the inclusion criteria, and four broad areas were identified: (i) Mental health impacts of tornadoes; (ii) Risk factors; (iii) Protective factors; and (iv) Mental health interventions. These findings indicate that tornadoes can have a significant impact on mental health in communities exposed (e.g., post-traumatic stress disorder, depression, anxiety) from months to years after a tornado. Studies have found increases in adverse mental health in both adult and pediatric populations. A number of risk factors were found to contribute to negative mental health, specifically demographics, post-tornado stressors, and prior exposure to trauma. Furthermore, a variety of protective factors were found to increase positive mental health outcomes. These included having access to physical, social, and psychological resources. Together, these findings can serve as an important resource for future mental health interventions in communities experiencing tornadoes. We also identified a need for future post-tornado mental health research among diverse and socially disadvantaged populations, including older adults, mobile/manufactured residents, people with disabilities, and the LGBTQ populations. Future studies examining these areas can help to identify factors and resources to protect individuals from negative consequences after tornado-related adversity and further develop disaster resilience systems.
Supplementary Materials
The following supporting information can be downloaded at: https://www.mdpi.com/article/10.3390/ijerph192113747/s1 , Table S1: Assessment of the Risk of bias, and Table S2: Supporting Information: Study characteristics and overview.
Funding Statement
This research received no external funding.
Author Contributions
The authors contributed as follows: conceptualization, S.L. and J.M.F.; methodology, S.L. and J.M.F.; writing, review, and editing, S.L. and J.M.F. All authors have read and agreed to the published version of the manuscript.
Institutional Review Board Statement
Not applicable.
Informed Consent Statement
Data availability statement, conflicts of interest.
The authors declare no conflict of interest.
Publisher’s Note: MDPI stays neutral with regard to jurisdictional claims in published maps and institutional affiliations.

- TORNADO PREDICTION.
- TORNADO DETECTION.
- TORNADO WARNING DECISION.
- WARNING DISSEMINATION.
- Understanding.
- Confirmation.
- Personalization of risk.
- Action necessary and feasible.
- NEXT STEPS.
Aguirre , B. E. , 1988 : The lack of warnings before the Saragosa tornado . Int. J. Mass Emerg. Disasters , 6 , 65 – 74 .
- Search Google Scholar
- Export Citation
Ahlborn , L. , and J. Franc , 2012 : Tornado hazard communication disparities among Spanish-speaking individuals in an English-speaking community . Prehosp. Disaster Med. , 26 , 1 – 5 .
Aldoorya , L. , J.-N. Kimb , and N. Tindall , 2010 : The influence of perceived shared risk in crisis communication: Elaborating the situational theory of publics . Public Relat. Rev. , 36 , 134 – 140 .
Ammons , P. , 2011 : Katelyn Cornwell and Harold ‘Butch’ Fitzgerald, Harvest tornado victims The Huntsville Times , 6 May 2011 . [Available online at http://blog.al.com/ht/2011/05/katelyn_cornwell_and_harold_bu.html .]
Andra , D. , E. Quoetone , and W. Bunting , 2002 : Warning decision making: The relative roles of conceptual models, technology, strategy, and forecaster expertise on 3 May 1999 . Wea. Forecasting , 17 , 559 – 566 .
Ashley , W. , 2007 : Spatial and temporal analysis of tornado fatalities in the United States: 1880–2005 . Wea. Forecasting , 22 , 1214 – 1228 .
Atwood , L. E. , and A. M. Major , 1998 : Exploring the “cry wolf” hypothesis . Int. J. Mass Emerg. Disasters , 16 , 279 – 302 .
Balluz , L. , L. Schieve , T. Holmes , S. Kiezak , and J. Malilay , 2000 : Predictors for people's response to a tornado warning, Arkansas, 1 March, 1997 . Disasters , 24 , 71 – 77 .
Barnes , L. , E. Gruntfest , M. Hayden , D. Schultz , and C. Benight , 2007 : False alarms and close calls: A conceptual model of warning accuracy . Wea. Forecasting , 22 , 1140 – 1147 .
Baumgart , L. A. , E. J. Bass , B. Philips , and K. Kloesel , 2008 : Emergency management decision making during severe weather . Wea. Forecasting , 23 , 1268 – 1279 .
Benjamin , S. , K. Brewster , R. Brümmer , B. Jewett , T. Schlatter , T. Smith , and P. Stamus , 1991 : An isentropic three-hourly data assimilation system using ACARS aircraft observations . Mon. Wea. Rev. , 119 , 888 – 906 .
Berry , L. , 1999 : Cyclone Rona: February 11, 1999 evacuation of Caravonica and Lake Placid report . Centre for Disaster Studies, James Cook University , 72 pp .
Biddle , M. D. , and D. R. Legates , 1999 : Warning response and risk behavior in the Oak Grove—Birmingham, Alabama, tornado of 08 April 1998 . Natural Hazards Research and Applications Information Center Quick Response Rep. 116 , 34 pp .
Bieringer , P. , and P. Ray , 1996 : A comparison of tornado warning lead times with and without NEXRAD Doppler radar . Wea. Forecasting , 11 , 47 – 52 .
Blanchard-Boehm , R. D. , 1998 : Understanding public response to increased risk from natural hazards: Application of the hazards risk communication framework . Int. J. Mass Emerg. Disasters , 16 , 247 – 278 .
Bodine , D. , M. Kumjian , R. Palmer , P. Heinselman , and A. Ryzhkov , 2013 : Tornado damage estimation using polarimetric radar . Wea. Forecasting , 28 , 139 – 158 .
Brewster , K. , J. Brotzge , K. Thomas , Y. Wang , M. Xue , J. Gao , D. Weber , and K. Howe , 2008 : High resolution assimilation in CASA and NEXRAD radar data in near real-time: Results from spring 2007 and plans for spring 2008 . Preprints, 12th Conf. on IOAS-AOLS , New Orleans, LA, Amer. Meteor. Soc., 15B.7. [Available online at https://ams.confex.com/ams/88Annual/techprogram/paper_132983.htm .]
Breznitz , S. , 1984 : Cry Wolf: The Psychology of False Alarms . Lawrence Earlbaum Associates , 265 pp .
Brooks , H. , 2004 : Tornado-warning performance in the past and future: A perspective from signal detection theory . Bull. Amer. Meteor. Soc. , 85 , 837 – 843 .
Brooks , H. , and C. Doswell , 2001 : Normalized damage from major tornadoes in the United States: 1890–1999 . Wea. Forecasting , 16 , 168 – 176 .
Brooks , H. , C. Doswell , and J. Cooper , 1994 : On the environments of tornadic and nontornadic mesocyclones . Wea. Forecasting , 9 , 606 – 618 .
Brotzge , J. , and S. Erickson , 2009 : NWS tornado warnings with zero or negative lead times . Wea. Forecasting , 24 , 140 – 154 .
Brotzge , J. , and S. Erickson , 2010 : Tornadoes without NWS warning . Wea. Forecasting , 25 , 159 – 172 .
Brotzge , J. , K. Hondl , B. Philips , L. Lemon , E. Bass , D. Rude , and D. Andra Jr. , 2010 : Evaluation of distributed collaborative adaptive sensing for detection of low-level circulations and implications for severe weather warning operations . Wea. Forecasting , 25 , 173 – 189 .
Brotzge , J. , S. Erickson , and H. Brooks , 2011 : A 5-yr climatology of tornado false alarms . Wea. Forecasting , 26 , 534 – 544 .
Brown , R. , W. Bumgarner , K. Crawford , and D. Sirmans , 1971 : Preliminary Doppler velocity measurements in a developing radar hook echo . Bull. Amer. Meteor. Soc. , 52 , 1186 – 1188 .
Brown , R. , V. Wood , and T. Barker , 2002 : Improved detection using negative elevation angles for mountaintop WSR-88Ds: Simulation of KMSX near Missoula, Montana . Wea. Forecasting , 17 , 223 – 237 .
Brown , R. , T. Niziol , N. Donaldson , P. Joe , and V. Wood , 2007 : Improved detection using negative elevation angles for mountaintop WSR-88Ds. Part III: Simulations of shallow convective activity over and around Lake Ontario . Wea. Forecasting , 22 , 839 – 852 .
Brown , S. , P. Archer , E. Kruger , and S. Mallonee , 2002 : Tornado-related deaths and injuries in Oklahoma due to the 3 May 1999 tornadoes . Wea. Forecasting , 17 , 343 – 353 .
Bryan , G. , and H. Morrison , 2012 : Sensitivity of a simulated squall line to horizontal resolution and parameterization of microphysics . Mon. Wea. Rev. , 140 , 202 – 225 .
Bryan , G. , J. Wyngaard , and J. Fritsch , 2003 : Resolution requirements for the simulation of deep moist convection . Mon. Wea. Rev. , 131 , 2394 – 2416 .
Carbin , G. , cited 2012 : Latest U.S. tornado statistics . Storm Prediction Center . [Available online at www.spc.noaa.gov/climo/online/monthly/newm.html .]
Clark , A. , W. Gallus , M. Xue , and F. Kong , 2010 : Convection-allowing and convection-parameterizing ensemble forecasts of a mesoscale convective vortex and associated severe weather environment . Wea. Forecasting , 25 , 1052 – 1081 .
Clark , A. , J. Kain , P. Marsh , J. Correia Jr. , M. Xue , and F. Kong , 2012 : Forecasting tornado pathlengths using a three-dimensional object identification algorithm applied to convection-allowing forecasts . Wea. Forecasting , 27 , 1090 – 1113 .
Cola , R. M. , 1996 : Responses of Pampanga households to lahar warnings: Lessons from two villages in the Pasig-Potrero River watershed . Fire and Mud: Eruptions and Lahars of Mount Pinatubo, Philippines , C. G. Newhall and R. S. Punongbayan , Eds ., University of Washington Press , 141 – 149 .
Coleman , T. , K. Knupp , J. Spann , J. Elliott , and B. Peters , 2011 : The history (and future) of tornado warning dissemination in the United States . Bull. Amer. Meteor. Soc. , 92 , 567 – 582 .
Crum , T. , and R. Alberty , 1993 : The WSR-88D and the WSR-88D Operational Support Facility . Bull. Amer. Meteor. Soc. , 74 , 1669 – 1687 .
Crum , T. , R. Alberty , and D. Burgess , 1993 : Recording, archiving, and using WSR-88D data . Bull. Amer. Meteor. Soc. , 74 , 645 – 653 .
Crum , T. , R. Saffle , and J. Wilson , 1998 : An update on the NEXRAD program and future WSR-88D support to operations . Wea. Forecasting , 13 , 253 – 262 .
Dabberdt , W. , and Coauthors , 2005 : Multifunctional mesoscale observing networks . Bull. Amer. Meteor. Soc. , 86 , 961 – 982 .
Davies , J. , 2004 : Estimations of CIN and LFC associated with tornadic and nontornadic supercells . Wea. Forecasting , 19 , 714 – 726 .
Dawson , D. , M. Xue , J. Milbrandt , and M. Yau , 2010 : Comparison of evaporation and cold pool development between single-moment and multimoment bulk microphysics schemes in idealized simulations of tornadic thunderstorms . Mon. Wea. Rev. , 138 , 1152 – 1171 .
Dawson , D. , L. Wicker , E. Mansell , and R. Tanamachi , 2012 : Impact of the environmental low-level wind profile on ensemble forecasts of the 4 May 2007 Greensburg, Kansas, tornadic storm and associated mesocyclones . Mon. Wea. Rev. , 140 , 696 – 716 .
de Elía , R. , and I. Zawadzki , 2001 : Optimal layout of a bistatic radar network . J. Atmos. Oceanic Technol. , 18 , 1184 – 1194 .
Diggory , J. , 1956 : Some consequences of proximity to a disease threat . Sociometry , 19 , 47 – 53 .
DiGiovanni , C. , B. Reynolds , R. Harwell , and E. Stonecipher , 2002 : A prospective study of the reactions of residents of an American community to a bioterrorist attack . Defense Threat Reduction Agency .
Dixon , E. , J. Sisskind , and S. Israel , 2012 : Helios: Real-time adaptive weather surveillance system using integrated camera networks and enhanced geospatial processing algorithms . Preprints, 16th Symp. Meteor. Observation Instrumentation , New Orleans, LA, Amer. Meteor. Soc., 230. [Available online at https://ams.confex.com/ams/92Annual/webprogram/Paper202905.html .]
Donner , W. , 2007 : The political ecology of disaster: An analysis of factors influencing U.S. tornado fatalities and injuries, 1998–2000 . Demography , 44 , 669 – 685 .
Donner , W. , 2008 : Decision making as community adaptation: A case study of emergency managers in Oklahoma . Disasters , 32 , 292 – 302 .
Donner , W. , and H. Rodríguez , 2008 : Population composition, migration, and inequality: The influence of demographic changes on disaster risk and vulnerability . Soc. Forces , 87 , 1089 – 1114 .
Donner , W. , H. Rodríguez , and W. Diaz , 2012 : Tornado warnings in three southern states: A qualitative analysis of public response patterns . J. Homeland Secur. Emerg. Manage. , 9 , doi:10.1515/1547-7355.1955 .
Doswell , C. , A. Moller , and H. Brooks , 1999 : Storm spotting and public awareness since the first tornado forecasts of 1948 . Wea. Forecasting , 14 , 544 – 557 .
Dow , K. , and S. Cutter , 1998 : Crying wolf: Repeat responses to hurricane evacuation orders . Coastal Manage. , 26 , 237 – 252 .
Drabek , T. , 1969 : Social processes in disaster: Family evacuation . Soc. Probl. , 16 , 336 – 349 .
Drabek , T. , 1994 : Disaster evacuation and the tourist industry . Natural Hazards Research and Applications Information Center, Institute of Behavioral Science, University of Colorado , 268 pp .
Edwards , M. L. , 1993 : Social location and self-protective behavior: Implications for earthquake preparedness . Int. J. Mass Emerg. Disasters , 11 , 293 – 303 .
Egentowich , J. M. , M. L. Kaplan , Y.-L. Lin , and A. J. Riordan , 2000a : Mesoscale simulations of dynamical factors discriminating between a tornado outbreak and non-event over the southeast US. Part I: 84–48 hour precursors . Meteor. Atmos. Phys. , 74 , 129 – 157 .
Egentowich , J. M. , M. L. Kaplan , Y.-L. Lin , and A. J. Riordan , 2000b : Mesoscale simulations of dynamical factors discriminating between a tornado outbreak and non-event over the southeast US. Part II: 48–6 hour precursors . Meteor. Atmos. Phys. , 74 , 159 – 187 .
Egentowich , J. M. , M. L. Kaplan , Y.-L. Lin , and A. J. Riordan , 2000c : Mesoscale simulations of dynamical factors discriminating between a tornado outbreak and non-event over the southeast US. Part III: 6 hour precursors . Meteor. Atmos. Phys. , 74 , 189 – 215 .
Erickson , S. , and H. Brooks , 2006 : Lead time and time under tornado warnings: 1986–2004 . Preprints, 23rd Conf. on Severe Local Storms , St. Louis, MO, Amer. Meteor. Soc., 11.5. [Available online at http://ams.confex.com/ams/23SLS/techprogram/paper_115194.htm .]
Farley , J. , H. Barlow , M. Finkelstein , and L. Riley , 1993 : Earthquake hysteria, before and after: A survey and follow-up on public response to the Browning forecast . Int. J. Mass Emerg. Disasters , 11 , 305 – 321 .
Fierro , A. , E. Mansell , C. Ziegler , and D. MacGorman , 2012 : Application of a lightning data assimilation technique in the WRF-ARW model at cloud-resolving scales for the tornado outbreak of 24 May 2011 . Mon. Wea. Rev. , 140 , 2609 – 2627 .
Flynn , C. , 1979 : Three Mile Island telephone survey: Preliminary report on procedures and findings . Mountain West Research Inc. Tech. Rep. , 90 pp .
Foster , H. D. , 1980 : Disaster Planning: The Preservation of Life and Property . Springer-Verlag , 255 pp .
Frame , J. , and P. Markowski , 2010 : Numerical simulations of radiative cooling beneath the anvils of super-cell thunderstorms . Mon. Wea. Rev. , 138 , 3024 – 3047 .
Frazier , K. , 1979 : The Violent Face of Nature: Severe Phenomena and Natural Disasters . William Morrow & Co. , 386 pp .
Friday , E. , 1994 : The modernization and associated restructuring of the National Weather Service: An overview . Bull. Amer. Meteor. Soc. , 75 , 43 – 52 .
Galway , J. , 1975 : Relationship of tornado deaths to severe weather watch areas . Mon. Wea. Rev. , 103 , 737 – 741 .
Golden , J. H. , and C. R. Adams , 2000 : The tornado problem: Forecast, warning, and response . Nat. Hazards Rev. , 1 , 107 – 118 .
Gusmano , M. K. , and V. G. Rodwin , 2006 : The elderly and social isolation . Testimony to Committee on Aging, New York City Council , 6 pp. [Available online at http://wagner.nyu.edu/faculty/testimony/rodwinNycCouncil021106.pdf .]
Haas , J. , H. Cochrane , and D. G. Eddy , 1977 : Consequences of a cyclone on a small city . Ekistics , 44 , 45 – 50 .
Hammer , B. , and T. Schmidlin , 2002 : Response to warnings during the 3 May 1999 Oklahoma City tornado: Reasons and relative injury rates . Wea. Forecasting , 17 , 577 – 581 .
Hansson , R. , D. Noulles , and S. Bellovich , 1982 : Knowledge, warning and stress . Environ. Behav. , 14 , 171 – 185 .
Heath , S. , 1999 : Animal Management in Disasters . Mosby, Inc. , 320 pp .
Heinselman , P. , D. LaDue , and H. Lazrus , 2012 : Exploring impacts of rapid-scan radar data on NWS warning decisions . Wea. Forecasting , 27 , 1031 – 1044 .
Heinselman , P. , D. LaDue , D. M. Kingfield , R. Hoffman , and B. W. MacAloney II , 2013 : Simulated NWS tornado warning decisions using rapid-scan radar data . Preprints, 29th Conf. Environ. Info. Processing Technologies , Austin, TX, Amer. Meteor. Soc., 8.3. [Available at https://ams.confex.com/ams/93Annual/webprogram/Paper219547.html .]
Hodge , D. , V. Sharp , and M. Marts , 1981 : Contemporary responses to volcanism: Case studies from the Cascades and Hawaii . Volcanic Activity and Human Ecology , P. D. Sheets and D. K. Grayson , Eds ., Academic Press , 221 – 248 .
Janis , I. L. , 1962 : Psychological effects of warnings . Man and Society in Disaster , G. Baker and D. Chapman , Eds ., Basic Books , 55 – 91 .
Jung , Y. , M. Xue , G. Zhang , and J. Straka , 2008 : Assimilation of simulated polarimetric radar data for a convective storm using the ensemble Kalman filter. Part II: Impact of polarimetric data on storm analysis . Mon. Wea. Rev. , 136 , 2246 – 2260 .
Junyent , F. , and V. Chandrasekar , 2009 : Theory and characterization of weather radar networks . J. Atmos. Oceanic Technol. , 26 , 474 – 491 .
Kalnay , E. , 2003 : Atmospheric Modeling, Data Assimilation and Predictability . Cambridge University Press , 341 pp .
Kelleher , K. , and Coauthors , 2007 : Project CRAFT: A real-time delivery system for NEXRAD level II data via the Internet . Bull. Amer. Meteor. Soc. , 88 , 1045 – 1057 .
Keller , C. , M. Siegrist , and H. Gutscher , 2006 : The role of the affect and availability heuristics in risk communication . Risk Anal. , 26 , 631 – 639 .
Kirschenbaum , A. , 1992 : Warning and evacuation during a mass disaster: A multivariate decision making model . Int. J. Mass Emerg. Disasters , 10 , 91 – 114 .
Kupec , R. J. , 2008 : Tuning in: Weather radios for those most at risk . J. Emerg. Manage. , 6 , 51 – 56 .
Kurdzo , J. , and R. Palmer , 2012 : Objective optimization of weather radar networks for low-level coverage using a genetic algorithm . J. Atmos. Oceanic Technol. , 29 , 807 – 821 .
LaDue , D. , P. Heinselman , and J. Newman , 2010 : Strengths and limitations of current radar systems for two stakeholder groups in the southern plains . Bull. Amer. Meteor. Soc. , 91 , 899 – 910 .
Lakshmanan , V. , and T. Smith , 2010 : An objective method of evaluating and devising storm tracking algorithms . Wea. Forecasting , 25 , 721 – 729 .
Lakshmanan , V. , T. Smith , K. Hondl , G. J. Stumpf , and A. Witt , 2006 : A real-time, three dimensional, rapidly updating, heterogeneous radar merger technique for reflectivity, velocity and derived products . Wea. Forecasting , 21 , 802 – 823 .
Lakshmanan , V. , T. Smith , G. Stumpf , and K. Hondl , 2007 : The Warning Decision Support System–Integrated Information . Wea. Forecasting , 22 , 596 – 612 .
Lardry , T. , and G. Rogers , 1982 : Warning confirmation and dissemination . Center for Social and Urban Research, University of Pittsburgh .
Lazo , J. K. , 2012 : One economist's entreaty for increased research on weather risk communication . Wea. Climate Soc. , 4 , 233 – 235 .
Leik , R. , T. Carter , and J. Clark , 1981 : Community response to natural hazard warning . U.S. Dept. of Commerce , 77 pp. [NTIS PB82-111287]
Leone , D. A. , R. M. Endlich , J. Petričeks , R. T. H. Collis , and J. R. Porter , 1989 : Meteorological considerations used in planning the NEXRAD network . Bull. Amer. Meteor. Soc. , 70 , 4 – 13 .
Li , J. , 1991 : Social responses to the Tangshan earthquake prediction . Preprints, UCLA Int. Conf. on the Impact of Natural Disasters , Los Angeles, CA, University of California, Los Angeles, CA. [Available online at http://udspace.udel.edu/handle/19716/537 .]
Lindell , M. K. , and R. W. Perry , 1983 : Nuclear power plant emergency warnings: How would the public respond? Nuclear News , February , 49 – 57 .
Lindell , M. K. , and R. W. Perry , 1992 : Behavioral Foundations of Community Emergency Planning . Hemisphere Publishing Corporation , 630 pp .
Lipkus , I. , and J. G. Hollands , 1999 : The visual communication of risk . J. Natl. Cancer Inst. Monogr. , 25 , 149 – 163 .
Maddox , R. , and C. Crisp , 1999 : The Tinker AFB tornadoes of March 1948 . Wea. Forecasting , 14 , 492 – 499 .
Mahale , V. , J. Brotzge , and H. Bluestein , 2012 : The analysis of vortices embedded within a quasi-linear convective system using X-band polarimetric radar . Wea. Forecasting , 27 , 1520 – 1537 .
Mahoney , B. , S. Drobot , P. Pisano , B. McKeever , and J. O'Sullivan , 2010 : Vehicles as mobile weather observation systems . Bull. Amer. Meteor. Soc. , 91 , 1179 – 1182 .
Manning , M. , 2007 : The effectiveness of NOAA weather radios as an all-hazards alert method in eastern Loudon County, Virginia . J. Emerg. Manage. , 17 , 343 – 353 .
Markowski , P. , 2002 : Hook echoes and rear-flank downdrafts: A review . Mon. Wea. Rev. , 130 , 852 – 876 .
Markowski , P. , and Y. Richardson , 2009 : Tornadogenesis: Our current understanding, forecasting considerations, and questions to guide future research . Atmos. Res. , 93 , 3 – 10 .
Markowski , P. , and N. Dotzek , 2011 : A numerical study of the effects of orography on supercells . Atmos. Res. , 100 , 457 – 478 .
Martin , W. , and M. Xue , 2006 : Sensitivity analysis of convection of the 24 May 2002 IHOP case using very large ensembles . Mon. Wea. Rev. , 134 , 192 – 207 .
Maximuk , L. , and M. Hudson , 2012 : Impact based warning: Experimental product . NWS Central Region presentation. [Available online at www.norman.noaa.gov/nsww/wp-content/uploads/2012/03/Hudson_NSWW2012.pdf .]
McCarthy , D. , 2002 : The role of ground-truth reports in the warning decision-making process during the 3 May 1999 Oklahoma tornado outbreak . Wea. Forecasting , 17 , 647 – 649 .
McDavid , J. , and M. Marai , 1968 : Social Psychology . Harper and Row , 479 pp .
McLaughlin , D. , and Coauthors , 2009 : Short-wavelength technology and the potential for distributed networks of small radar systems . Bull. Amer. Meteor. Soc. , 90 , 1797 – 1817 .
Mead , C. , 1997 : The discrimination between tornadic and nontornadic supercell environments: A forecasting challenge in the Southern United States . Wea. Forecasting , 12 , 379 – 387 .
Mercer , A. , C. Shafer , C. Doswell , L. Leslie , and M. Richman , 2009 : Objective classification of tornadic and nontornadic severe weather outbreaks . Mon. Wea. Rev. , 137 , 4355 – 4368 .
Mileti , D. S. , 1999 : Disasters by Design . Joseph Henry Press , 376 pp .
Mileti , D. S. , and J. H. Sorensen , 1990 : Communication of emergency public warnings: A social science perspective and state-of-the-art assessment . Oak Ridge National Laboratory Tech. Rep. ORNL-6609 , 166 pp .
Mileti , D. S. , and C. Fitzpatrick , 1993 : Great Earthquake Experiment: Risk Communication and Public Action . Westview Press , 149 pp .
Mileti , D. S. , and J. D. Darlington , 1995 : Societal response to revised earthquake probabilities in the San Francisco Bay area . Int. J. Mass Emerg. Disasters , 13 , 119 – 145 .
Mileti , D. S. , J. R. Hutton , and J. H. Sorensen , 1981 : Earthquake Prediction Response and Options for Public Policy . Institute of Behavioral Science, University of Colorado , 152 pp .
Miller , M. , V. Lakshmanan , and T. Smith , 2013 : An automated method for depicting mesocyclone paths and intensities . Wea. Forecasting , 28 , 570 – 585 .
Miller , R. , and C. Crisp , 1999a : Events leading to the establishment of the United States Air Force Severe Weather Warning Center in February 1951 . Wea. Forecasting , 14 , 500 – 506 .
Miller , R. , and C. Crisp , 1999b : The first operational tornado forecast twenty million to one . Wea. Forecasting , 14 , 479 – 483 .
Miller , R. , M. Barth , L. Benjamin , R. Artz , and W. Pendergrass , 2007 : MADIS support for UrbaNet . Preprints, 14th Symp. on Meteorological Observation and Instrumentation , San Antonio, TX, Amer. Meteor. Soc., JP2.5. [Available online at https://ams.confex.com/ams/87ANNUAL/webprogram/Paper119116.html .]
Minciardi , R. , R. Sacile , and F. Siccardi , 2003 : Optimal planning of a weather radar network . J. Atmos. Oceanic Technol. , 20 , 1251 – 1263 .
Mitchell , E. , S. Vasiloff , G. Stumpf , A. Witt , M. Eilts , J. T. Johnson , and K. Thomas , 1998 : The National Severe Storms Laboratory tornado detection algorithm . Wea. Forecasting , 13 , 352 – 366 .
Mitchem , J. D. , 2003 : An analysis of the September 20, 2002, Indianapolis tornado: Public response to a tornado warning and damage assessment difficulties . Natural Hazards Research and Applications Information Center Quick Response Rep. 161 , 55 pp. [Available online at www.colorado.edu/hazards/research/qr/qr161/qr161.pdf .]
Moller , A. , 1978 : The improved NWS storm spotters' training program at Ft. Worth, TX . Bull. Amer. Meteor. Soc. , 59 , 1574 – 1582 .
Moore , G. , 1965 : Cramming more components onto integrated circuits . Electronics , 38 , 114 – 117 .
Moore , T. , and R. Dixon , 2012 : Tropical cyclone-tornado casualties . Nat. Hazards , 61 , 621 – 634 .
Morss , R. , J. K. Lazo , and J. L. Demuth , 2010 : Examining the use of weather forecasts in decision scenarios: Results from a US survey with implications for uncertainty communication . Meteor. Appl. , 17 , 149 – 162 .
Nagarajan , M. , D. Shaw , and P. Albores , 2012 : Disseminating a warning message to evacuate: A simulation study of the behaviour of neighbours . Eur. J. Oper. Res. , 220 , 810 – 819 .
National Research Council , 1995 : Toward a New National Weather Service: Assessment of NEXRAD Coverage and Associated Weather Services . National Academies Press , 104 pp .
National Research Council , 2009 : Observing Weather and Climate from the Ground Up: A Nationwide Network of Networks . National Academies Press , 234 pp .
NOAA , 2011a : Service assessment: The historic tornadoes of April 2011 . NOAA NWS Rep. , 73 pp. [Available online at www.nws.noaa.gov/os/assessments/pdfs/historic_tornadoes.pdf .]
NOAA , 2011b : Tornado warnings (nation) . NOAA NWS , 10 pp. [Available online at https://verification.nws.noaa.gov/services/gpra/NWS_GPRA_Metrics.pdf .]
Ortega , K. L. , T. M. Smith , K. L. Manross , K. A. Scharfenberg , A. Witt , A. G. Kolodziej , and J. J. Gourley , 2009 : The Severe Hazards Analysis and Verification Experiment . Bull. Amer. Meteor. Soc. , 90 , 1519 – 1530 .
Ostby , F. , 1999 : Improved accuracy in severe storm forecasting by the Severe Local Storms Unit during the last 25 years: Then versus now . Wea. Forecasting , 14 , 526 – 543 .
Otkin , J. , D. Hartung , D. Turner , R. Petersen , W. Feltz , and E. Janzon , 2011 : Assimilation of surface-based boundary layer profiler observations during a cool-season weather event using an Observing System Simulation Experiment. Part I: Analysis impact . Mon. Wea. Rev. , 139 , 2309 – 2326 .
Park , S. , and L. Xu , 2009 : Data Assimilation for Atmospheric, Oceanic, and Hydrologic Applications . Springer , 495 pp .
Pearson , A. , and S. Weiss , 1979 : Some trends in forecast skill at the National Severe Storms Forecast Center . Bull. Amer. Meteor. Soc. , 60 , 319 – 326 .
Peguero , A. A. , 2006 : Latino disaster vulnerability: The dissemination of hurricane mitigation Information among Florida's homeowners . Hisp. J. Behav. Sci. , 28 , 5 – 22 .
Perry , R. W. , 1979 : Evacuation decision-making in natural disasters . Int. J. Mass Emerg. Disasters , 4 , 25 – 38 .
Perry , R. W. , 1983 : Population evacuation in volcanic eruptions, floods, and nuclear power plant accidents: Some elementary comparisons . J. Community Psychol. , 11 , 36 – 47 .
Perry , R. W. , 1987 : Disaster preparedness and response among minority citizens . Sociology of Disasters , R. R. Dynes , B. DeMarchi , and C. Pelanda , Eds ., Franco Angeli Libri , 135 – 151 .
Perry , R. W. , and M. Greene , 1983 : Citizen Response to Volcanic Eruptions: The Case of Mt. St. Helens . Irvington Publishers , 145 pp .
Perry , R. W. , and M. Lindell , 1986 : Twentieth century volcanicity at Mt. St. Helens: The routinization of life near an active volcano . School of Public Affairs, Arizona State University , Tech. Rep. 179 pp .
Perry , R. W. , and H. Hirose , 1991 : Volcano Management in the United States and Japan . Jai Press , 230 pp .
Perry , R. W. , H. Hirose , and M. R. Greene , 1981 : Evacuation Planning in Emergency Management . Lexington Books , 199 pp .
Pfister , N. , 2002 : Community response to flood warnings: The case of an evacuation from Grafton, March 2001 . Aust. J. Emerg. Manag. , 17 , 19 – 29 .
Polger , P. , B. Goldsmith , R. Przywarty , and J. Bocchieri , 1994 : National Weather Service Warning performance based on the WSR-88D . Bull. Amer. Meteor. Soc. , 75 , 203 – 214 .
Powell , S. W. , and H. D. O'Hair , 2008 : Communicating weather information to the public: People's reactions and understandings of weather information and terminology . Preprints, Third Symp. on Policy and Socio-Economic Research , New Orleans, LA, Amer. Meteor. Soc., P1.3. [Available at http://ams.confex.com/ams/pdfpapers/132939.pdf .]
Quarantelli , E. L. , 1980 : Evacuation behavior and problems: Findings and implications from the research literature . Disaster Research Center, The Ohio State University , Miscellaneous Rep. 27 , 214 pp .
Quoetone , E. , J. Boettcher , and C. Spannagle , 2009 : How did that happen? A look at factors that go into forecaster warning decisions . Extended Abstracts, 34th Annual Meeting , Norfolk, VA , National Weather Association . [Available online at www.nwas.org/meetings/nwa2009/ .]
Rasmussen , E. , J. Straka , R. Davies-Jones , C. Doswell , F. Carr , M. Eilts , and D. MacGorman , 1994 : Verification of the Origins of Rotation in Tornadoes Experiment: VORTEX . Bull. Amer. Meteor. Soc. , 75 , 995 – 1006 .
Ray , P. , and K. Sangren , 1983 : Multiple-Doppler radar network design . J. Climate Appl. Meteor. , 22 , 1444 – 1454 .
Richter , H. , and L. Bosart , 2002 : The suppression of deep moist convection near the southern Great Plains dryline . Mon. Wea. Rev. , 130 , 1665 – 1691 .
Rogers , G. , and J. Nehnevajsa , 1987 : Warning human populations of technological hazards . Radiological Accidents: Perspectives and Emergency , C. Chester and K. S. Grant , Eds ., American Nuclear Society , 357 – 362 .
Rogers , G. , and J. H. Sorensen , 1991 : Diffusion of emergency warning: Comparing empirical and simulation results . Risk Anal. , 11 , 117 – 134 .
Ryzhkov , A. , T. Schuur , D. Burgess , and D. Zrnic , 2005 : Polarimetric tornado detection . J. Appl. Meteor. , 44 , 557 – 570 .
Saarinen , T. , V. Baker , R. Durrenberger , and T. Maddock Jr. , 1984 : The Tucson, Arizona, Flood of October 1983 . National Academy Press , 112 pp .
Scanlon , J. , and A. Frizzell , 1979 : Old theories don't apply: Implications of communication in crisis . Disasters , 3 , 315 – 319 .
Schenkman , A. , M. Xue , A. Shapiro , K. Brewster , and J. Gao , 2011 : Impact of CASA radar and Oklahoma Mesonet data assimilation on the analysis and prediction of tornadic mesovortices in an MCS . Mon. Wea. Rev. , 139 , 3422 – 3445 .
Schmidlin , T. W. , and P. S. King , 1997 : Risk factors for death in the 1 March 1997 Arkansas tornadoes . Natural Hazards Research and Applications Information Center, Quick Response Rep. QR98. [Available online at www.colorado.edu/hazards/research/qr/qr98.html .]
Schultz , C. , and M. Askelson , 2012 : Vertical variations of boundary layer potential buoyancy in tornadic and nontornadic near-storm environments . Wea. Forecasting , 27 , 1489 – 1506 .
Schultz , D. , E. Gruntfest , M. Hayden , C. Benight , S. Drobot , and L. Barnes , 2010 : Decision making by Austin, Texas, residents in hypothetical tornado scenarios . Wea. Climate Soc. , 2 , 249 – 254 .
Schultz , L. , and D. Cecil , 2009 : Tropical cyclone tornadoes, 1950–2007 . Mon. Wea. Rev. , 137 , 3471 – 3484 .
Shafer , C. M. , A. E. Mercer , C. A. Doswell , M. B. Richman , and L. M. Leslie , 2009 : Evaluation of WRF forecasts of tornadic and nontornadic outbreaks when initialized with synoptic-scale input . Mon. Wea. Rev. , 137 , 1250 – 1271 .
Shafer , C. M. , A. E. Mercer , L. M. Leslie , M. B. Richman , and C. A. Doswell III , 2010 : Evaluation of WRF model simulations of tornadic and nontornadic outbreaks occurring in the spring and fall . Mon. Wea. Rev. , 138 , 4098 – 4119 .
Sherman-Morris , K. , 2006 : Tornadoes, television, and trust: A closer look at the influence of the local weather forecaster during severe weather . Environ. Hazards , 6 , 201 – 210 .
Sherman-Morris , K. , 2010 : Tornado warning dissemination and response at a university campus . Nat. Hazards , 52 , 623 – 638 .
Simmons , K. , and D. Sutter , 2005 : WSR-88D radar, tornado warnings, and tornado casualties . Wea. Forecasting , 20 , 301 – 310 .
Simmons , K. , and D. Sutter , 2008 : Tornado warnings, lead times, and tornado casualties: An empirical investigation . Wea. Forecasting , 23 , 246 – 258 .
Simmons , K. , and D. Sutter , 2009 : False alarms, tornado warnings, and tornado casualties . Wea. Climate Soc. , 1 , 38 – 53 .
Simmons , K. , D. Sutter , and R. Pielke , 2013 : Normalized tornado damage in the United States: 1950–2011 . Environ. Hazards , 12 , 132 – 147 , doi:10.1080/17477891.2012.738642 .
Smith , P. , 1999 : Effects of imperfect storm reporting on the verification of weather warnings . Bull. Amer. Meteor. Soc. , 80 , 1099 – 1105 .
Smith , T. M. , and K. L. Elmore , 2004 : The use of radial velocity derivatives to diagnose rotation and divergence . Preprints, 11th Conf. on Aviation, Range, and Aerospace , Hyannis, MA, Amer. Meteor. Soc., P5.6. [Available online at https://ams.confex.com/ams/11aram22sls/techprogram/paper_81827.htm .]
Snook , N. , M. Xue , and Y. Jung , 2012 : Ensemble probabilistic forecasts of a tornadic mesoscale convective system from ensemble Kalman filter analyses using WSR-88D and CASA radar data . Mon. Wea. Rev. , 140 , 2126 – 2146 .
Sorensen , J. H. , 1982 : Evaluation of the emergency warning system at the Fort St. Vrain nuclear power plan . Oak Ridge National Laboratory Tech. Memo. ORNL/TM-8171 , 80 pp .
Sorensen , J. H. , and P. J. Gersmehl , 1980 : Volcanic hazard warning system: Persistence and transferability . Environ. Manage. , 4 , 125 – 136 .
Sorensen , J. H. , and D. S. Mileti , 1987 : Decision-making uncertainties in emergency warning system organizations . Int. J. Mass Emerg. Disasters , 5 , 33 – 61 .
Stallings , R. , 1990 : Media discourse and the social construction of risk . Soc. Probl. , 37 , 80 – 95 .
Stensrud , D. J. , and S. J. Weiss , 2002 : Mesoscale model ensemble forecasts of the 3 May 1999 tornado outbreak . Wea. Forecasting , 17 , 526 – 543 .
Stensrud , D. J. , J. Cortinas , and H. Brooks , 1997 : Discriminating between tornadic and nontornadic thunderstorms using mesoscale model output . Wea. Forecasting , 12 , 613 – 632 .
Stensrud , D. J. , and Coauthors , 2009 : Convective-scale warn-on-forecast system . Bull. Amer. Meteor. Soc. , 90 , 1487 – 1499 .
Stewart , T. R. , and C. M. Lusk , 1994 : Seven components of judgmental forecasting skill: Implications for research and the improvement of forecasts . J. Forecasting , 13 , 579 – 599 .
Stough , S. , E. Leitman , J. Peters , and J. Correia Jr. , 2012 : On the role of Storm Prediction Center products in decision making leading up to hazardous weather events . Preprints, 11th Annual AMS Student Conf. and Career Fair , New Orleans, LA, Amer. Meteor. Soc., S125. [Available online at https://ams.confex.com/ams/92Annual/webprogram/Paper204254.html .]
Stuart , N. , and Coauthors , 2006 : The future of humans in an increasingly automated forecast process . Bull. Amer. Meteor. Soc. , 87 , 1497 – 1502 .
Stumpf , G. , A. Witt , E. D. Mitchell , P. L. Spencer , J. T. Johnson , M. D. Eilts , K. W. Thomas , and D. W. Burgess , 1998 : The National Severe Storms Laboratory mesocyclone detection algorithm for the WSR-88D . Wea. Forecasting , 13 , 304 – 326 .
Sutter , D. , and S. Erickson , 2010 : The time cost of tornado warnings and the savings with storm-based warnings . Wea. Climate Soc. , 2 , 103 – 112 .
Sutter , D. , and K. Simmons , 2010 : Tornado fatalities and mobile homes in the United States . Nat. Hazards , 53 , 125 – 137 .
Sutter , D. , D. DeSilva , and J. Kruse , 2009 : An economic analysis of wind resistant construction . J. Wind Eng. Ind. Aerodyn. , 97 , 113 – 119 .
Tierney , K. , 1987 : Chemical emergencies, offsite exposures and organizational response . Natural Hazards Research and Applications Center, Institute of Behavioral Science, University of Colorado, Quick Response Rep. QR21 , 24 pp .
Trapp , R. , S. Tessendorf , E. Savageau Godfrey , and H. Brooks , 2005 : Tornadoes from squall lines and bow echoes. Part I: Climatological distribution . Wea. Forecasting , 20 , 23 – 34 .
Turner , R. H. , J. M. Nigg , D. H. Paz , and B. S. Young , 1979 : Earthquake threat: The human response in southern California . Institute for Social Science Research, University of California, Los Angeles , Tech. Rep. , 152 pp .
Unidata , cited 2012 : THREDDS . [Available online at www.unidata.ucar.edu/projects/THREDDS/ .]
Wakimoto , R. , and J. Wilson , 1989 : Non-supercell tornadoes . Mon. Wea. Rev. , 117 , 1113 – 1140 .
Wang , Y. , T.-Y. Yu , M. Yeary , A. Shapiro , S. Nemati , M. Foster , D. Andra Jr. , and M. Jain , 2008 : Tornado detection using a neuro–fuzzy system to integrate shear and spectral signatures . J. Atmos. Oceanic Technol. , 25 , 1136 – 1148 .
Whiton , R. C. , P. L. Smith , S. G. Bigler , K. E. Wilk , and A. C. Harbuck , 1998 : History of operational use of weather radar by U.S. weather services. Part II: Development of operational Doppler weather radars . Wea. Forecasting , 13 , 244 – 252 .
Wood , V. , R. Brown , and S. Vasiloff , 2003 : Improved detection using negative elevation angles for mountaintop WSR-88Ds. Part II: Simulations of the three radars covering Utah . Wea. Forecasting , 18 , 393 – 403 .
Wurman , J. , D. Dowell , Y. Richardson , P. Markowski , E. Rasmussen , D. Burgess , L. Wicker , and H. Bluestein , 2012 : The Second Verification of the Origins of Rotation in Tornadoes Experiment: VORTEX2 . Bull. Amer. Meteor. Soc. , 93 , 1147 – 1170 .
Zrnić , D. S. , and Coauthors , 2007 : Agile-beam phased array radar for weather observations . Bull. Amer. Meteor. Soc. , 88 , 1753 – 1766 .
Zubrick , S. , 2010 : Tornadoes: Understanding how they develop and providing early warning; Part III NWS . Hazards Caucus Alliance Briefing Series: Tornadoes; Understanding How They Develop and Providing Early Warning , Washington DC , American Geological Institute . [Available online at www.hazardscaucus.org/briefings/zubrick0710.pdf .]
Summary of the institutional and individual responses that comprise the tornado warning process.
- View raw image
- Download Powerpoint Slide
All Time | Past Year | Past 30 Days | |
---|---|---|---|
Abstract Views | 0 | 0 | 0 |
Full Text Views | 4177 | 917 | 78 |
PDF Downloads | 2725 | 501 | 35 |
Related Content
The impacts of interannual climate variability on the declining trend in terrestrial water storage over the tigris–euphrates river basin, evaluation of snowfall retrieval performance of gpm constellation radiometers relative to spaceborne radars, a 440-year reconstruction of heavy precipitation in california from blue oak tree rings, quantifying the role of internal climate variability and its translation from climate variables to hydropower production at basin scale in india, extreme convective rainfall and flooding from winter season extratropical cyclones in the mid-atlantic region of the united states.
- Previous Article
- Next Article
The Tornado Warning Process: A Review of Current Research, Challenges, and Opportunities
Displayed acceptance dates for articles published prior to 2023 are approximate to within a week. If needed, exact acceptance dates can be obtained by emailing [email protected] .
- Download PDF
With the unusually violent tornado season of 2011, there has been a renewed national interest, through such programs as NOAA's Weather Ready Nation initiative, to reevaluate and improve our tornado warning process. This literature review provides an interdisciplinary, end-to-end examination of the tornado warning process. Following the steps outlined by the Integrated Warning System, current research in tornado prediction and detection, the warning decision process, warning dissemination, and public response are reviewed, and some of the major challenges for improving each stage are highlighted. The progress and challenges in multi-day to short-term tornado prediction are discussed, followed by an examination of tornado detection, focused primarily upon the contributions made by weather radar and storm spotters. Next is a review of the warning decision process and the challenges associated with dissemination of the warning, followed by a discussion of the complexities associated with understanding public response. Finally, several research opportunities are considered, with emphases on understanding acceptable risk, greater community and personal preparation, and personalization of the hazard risk.
A review of the entire warning system, from prediction and detection to public response, reveals such fundamental needs as identifying acceptable risks, improving personal preparation, and personalizing warnings.
One of the scientific community's greatest achievements in meteorology during the twentieth century has been the development of a largely effective public tornado warning system. Between 1912 and 1936, tornadoes killed an average 260 persons per year, about 1.8 deaths per million people when normalized by population ( Brooks and Doswell 2001 ). Between 1975 and 2000, that number had declined to 54 deaths per year, or 0.12 deaths per million people in 2000 ( Brooks and Doswell 2001 ), a reduction of 93% from 1925. In 1986 the tornado warning lead time was approximately five minutes, with only 25% of tornadoes warned; by 2004, the mean lead time was 13 min, with about 75% of tornadoes warned ( Erickson and Brooks 2006 ).
Far from simple, the tornado warning process is a complex chain of events, encompassing institutional action and individual responses, that utilizes sensing technologies, conceptual models, numerical weather prediction (NWP), forecaster and emergency management (EM) decision making, warning dissemination technologies, and public experience and education ( Fig. 1 ). The sequential steps of this process—forecast, detection, warning decision, dissemination, and public response—are known as the Integrated Warning System (IWS; Leik et al. 1981 ; Doswell et al. 1999 ).
Citation: Bulletin of the American Meteorological Society 94, 11; 10.1175/BAMS-D-12-00147.1
- Download Figure
- Download figure as PowerPoint slide
This article reviews the end-to-end tornado warning process and related research, considers the challenges to improving the current system, and explores possible next steps. While this article cannot provide a completely comprehensive review of all research in each specific area, the goal is to provide a broad overview of the tornado warning process and a brief summary of the many avenues of research that could contribute to improvements in the current system.
The ability to predict a tornado's precise path and intensity days in advance could allow for evacuation to take place well ahead of storm development and the predeployment of assets needed to support emergency response and recovery. While restrained to less accurate forecasts by the inherent limitations imposed by atmospheric predictability, the last decade has seen a growing recognition of the connection between large-scale patterns and large-scale tornado outbreaks.
As high-resolution, convection-allowing (≤4-km grid resolution) NWP becomes more accurate at longer time scales, multivariate model output may be used to a greater extent in identifying and predicting tornado outbreak events. Using observational and modeling analysis, Egentowich et al. (2000a , b , c) identified a series of dynamic precursors during the 6–84 h preceding a major tornado outbreak. Shafer et al. (2009) found that Weather Research and Forecasting (WRF) model output could be used to discriminate between tornadic and nontornadic events up to three days in advance. Using WRF simulation output, Mercer et al. (2009) developed a statistical objective analysis technique to extract relevant predictive variables, yielding statistically significant accuracy scores >0.7 and skill scores >0.5 of these variables one day in advance of storm formation ( Shafer et al. 2010 ).
Ever faster computer processing, and increasing memory and storage capacities combined with advances in parallel computing and code efficiency now enable the routine use of mesoscale forecast ensembles at high-resolution hours or even days in advance. Furthermore, analysis of model ensembles provides insight into forecast uncertainty. Stensrud and Weiss (2002) demonstrated that even a relatively coarse (32-km inner grid), small six-member ensemble, while underdispersive, provided some statistical guidance in predicting the relative locations of expected severe weather 24 h in advance. Clark et al. (2010) have since shown that the use of convection-allowing resolutions improves the representation and prediction of severe weather features. As a predictive measure of storm severity, Clark et al. (2012) extracted proxy forecasts of tornado pathlengths from 36-h ensemble forecasts.
One official National Weather Service (NWS) product to alert local weather forecast offices, emergency personnel, and the public of favorable conditions for tornadoes to occur is the tornado watch . First issued by the Severe Local Storms Unit [SELS, now the Storm Prediction Center (SPC)] in 17 March 1952 ( Galway 1975 ), the tornado watch is a manually generated product, based upon NWP output and observations, and may be issued up to several hours in advance of initial convective initiation. The skill level of the tornado watch has continued to improve over the years with increased observations, refined conceptual models, and more accurate and higher-resolution NWP ( Pearson and Weiss 1979 ; Ostby 1999 ). Two additional, increasingly popular products issued by the SPC are the convective outlooks and mesoscale convective discussions (MCDs; Stough et al. 2012 ). Convective outlooks are issued up to eight days in advance, highlighting areas of the country with the potential for severe weather. MCDs are used to highlight general areas of concern, often issued just hours ahead of convective initiation or just prior to issuance of a watch. Both convective outlooks and MCDs are composed of a discussion briefing and visual map, and provide additional lead time and probabilistic information.
Currently, all official NWS tornado warnings are issued based upon “detections,” where an immediate tornado threat is observed either directly by spotters and media or inferred from observations (e.g., radar). However, as the accuracy and precision of short-term (0–3 h) storm predictions continue to improve, model output is expected to become an increasingly important basis upon which to issue NWS tornado warnings. This is the eventual goal of “warn on forecast” ( Stensrud et al. 2009 ), where NWS tornado warnings may be issued based not only on detected tornadoes or observed precursors, but also on model output. Utilizing model output as the basis for some warnings could theoretically extend lead time to tornadogenesis.
Significant advances in computer processing, the utilization of new types and greater numbers of real-time weather observations ( NRC 2009 ), and the development and adoption of new data assimilation (DA) techniques ( Kalnay 2003 ; Park and Xu 2009 ) are making warn on forecast a reality. Computer processing capabilities continue increasing at an exponential rate, as predicted by Moore's law ( Moore 1965 ). Faster processing permits higher-resolution NWP, which allows for the direct use of convective-resolving physics, bypassing less accurate parameterization schemes. The use of new observations, such as dual-polarimetric radar (e.g., Jung et al. 2008 ), wind and temperature profilers (e.g., Otkin et al. 2011 ), data from aircraft [e.g., Aircraft Communication, Addressing, and Reporting System (ACARS); Benjamin et al. 1991 ], lightning data ( Fierro et al. 2012 ), and new evolving mobile platforms (e.g., Mahoney et al. 2010 ) facilitates a more accurate, three-dimensional analysis of the initial conditions. Model initialization also has been made easier with greater access to real-time observations through the use of such systems as the Collaborative Radar Acquisition Field Test (CRAFT; Kelleher et al. 2007 ), the Meteorological Assimilation Data Ingest System (MADIS; Miller et al. 2007 ), and Thematic Real-time Environmental Distributed Data Services (THREDDS; Unidata 2012 ); see “Prediction challenges” for more information.
PREDICTION CHALLENGES
Several significant challenges remain to be addressed before routine 0–3-h tornado prediction can be realized. These needs include i) faster computer processing to permit even higher-resolution NWP and more robust ensemble systems; ii) the ability to enable real-time DA of even larger volumes of data; iii) greater numbers of observations at high spatial and temporal resolutions; and iv) the ability to predict marginal, less predictable events with greater accuracy and fewer false alarms. Model grid spacing is tightly coupled with the model physics; for example, Bryan et al. (2003) determined that model grid spacing on the order of 100 m is needed to fully resolve subgrid-scale turbulence. Parameterization schemes, such as cloud microphysics, convective, and planetary boundary layer schemes, fail to capture subgrid-scale processes which can lead to large sensitivities in storm-scale NWP results (e.g., Dawson et al. 2010 ; Bryan and Morrison 2012 ).
In a similar manner, storm-scale NWP is equally sensitive to model initialization and analysis. Numerical modeling of convective storms has shown sensitivity to model initialization of low-level thermodynamics ( Frame and Markowski 2010 ), low-level wind profiles ( Dawson et al. 2012 ), surface soil moisture ( Martin and Xue 2006 ), and orography ( Markowski and Dotzek 2011 ). Model assimilation sensitivity may be reduced by increasing the number and use of observations in critical areas ( Schenkman et al. 2011 ; Snook et al. 2012 ) and at critical times ( Richter and Bosart 2002 ). However, the ability to collect, quality control, and properly assimilate all the necessary data in real time at high resolutions is a significant challenge (e.g., Brewster et al. 2008 ). To address this issue, an optimally designed national observing network is needed to collect the necessary observations at the high resolutions required (e.g., low-level moisture and wind profiles; Dabberdt et al. 2005 ; National Research Council 2009 ).
Finally, while our ability to anticipate and predict significant events is relatively good with a POD of nearly 90% for tornado outbreaks ( Brotzge and Erickson 2009 ), the community faces significant challenges in predicting marginal and/or weakly forced tornado events. Brotzge and Erickson (2009) found the first tornado of the day, solitary tornado events, tornadoes from hurricanes, and weak (F0, F1) tornadoes had a much greater chance of not being warned. The FAR has been found to be highest for weakly forced and isolated events ( Brotzge et al. 2011 ). Nonsupercell tornadoes, such as from tropical storms ( Schultz and Cecil 2009 ; Moore and Dixon 2012 ) and squall lines ( Trapp et al. 2005 ), pose a significant difficulty for prediction because of their often transient nature. Among the greatest remaining challenges for tornado prediction are the ability to predict exactly when a tornado will initiate ( Markowski and Richardson 2009 ), to differentiate between tornadic and nontornadic supercells ( Brooks et al. 1994 ; Stensrud et al. 1997 ; Mead 1997 ; Davies 2004 ; Schultz and Askelson 2012 ), and to identify threatening nonsupercell tornadic storms ( Wakimoto and Wilson 1989 ).
Weather radar is the primary tool used by warning forecasters to identify areas of potential tornado development. Radar reflectivity provides forecasters with a clear view of tornadic features, such as the hook echo ( Markowski 2002 ), and Doppler radial velocity shows horizontal wind shear, sometimes an early indicator of tornado formation ( Brown et al. 1971 ). Radar polarimetric data provide storm microphysical information, such as hydrometeor type and shape, that can be used to identify areas of significant low-level wind shear (referred to as Z DR arcs) and tornado debris ( Ryzhkov et al. 2005 ; Bodine et al. 2013 ).
To better standardize weather radar coverage across the United States, the national Weather Surveillance Radar-1988 Doppler (WSR-88D) network [known as Next Generation Weather Radar (NEXRAD); Crum and Alberty 1993 ; Crum et al. 1993 , 1998 ] was deployed ( Whiton et al. 1998 ). The WSR-88D scanning geometry was designed to facilitate complete coverage between 610 m (2000 ft) and ~18 km (60,000 ft) AGL, with minimum height coverage at or below 610 m within a range of 102 km from radar ( Leone et al. 1989 ); the final network provided contiguous coverage across the United States at 3.05 km (10,000 ft) and above ( Crum and Alberty 1993 ). Specific radar site locations were chosen based primarily upon population distribution, severe weather climatology, topography, and proximity to other radars; most radars were sited to provide coverage over and slightly upwind of major metropolitan areas ( Leone et al. 1989 ). As of 2012, 160 WSR-88D (S band) systems comprised the NEXRAD network across the United States and territories.
NEXRAD deployment had an immediate and significant positive impact on tornado warning statistics ( Polger et al. 1994 ; NRC 1995 ). Bieringer and Ray (1996) found that the probability of detection (POD) increased by 10%–15% and that warning lead times increased by several minutes after installation of the WSR-88D network. Analyzing all tornadoes in the conterminous United States (CONUS) between 1986 and 1999, Simmons and Sutter (2005) estimated that the deployment of NEXRAD increased the percentage of tornadoes warned from 35.0% to 59.7%, increased the lead time from 5.3 to 9.5 min, reduced the false alarm ratio (FAR) from 78.6% to 76.0%, and reduced the number of expected fatalities and injuries by 45% and 40%, respectively. Smith (1999) , however, noted that verification procedures changed as the NEXRAD system was deployed, possibly accounting for some of the observed increase in the POD.
For enhanced tornado detection, automated detection algorithms, such as the WSR-88D mesocyclone ( Stumpf et al. 1998 ) and tornado detection algorithms (MDA and TDA, respectively; Mitchell et al. 1998 ), automatically identify radar-based tornado features and are displayed in real time within the Advanced Weather Interactive Processing System (AWIPS). Radar data can be combined with additional weather information to linearly project storm motion and extrapolate mesocyclone, tornado, and hail core movement (e.g., Smith and Elmore 2004 ; Lakshmanan et al. 2007 ; Wang et al. 2008 ; Ortega et al. 2009 ; Lakshmanan and Smith 2010 ; Miller et al. 2013 ).
Storm reports from individuals in the field can provide timely, critical information to warning officials. Trained “storm spotters” provide a valuable service to the NWS, EMs, and media by providing reliable, real-time information on storm evolution and tornado development ( Moller 1978 ; McCarthy 2002 ). As well documented by Doswell et al. (1999) , storm spotter networks were first organized during World War II largely to protect military installations. By the mid-1960s, spotter groups were organized more formally by the Weather Bureau for more general use under its SKYWARN program. With the advent of cell phone and embedded camera technology, widespread access to the Internet, television station helicopters, volunteer and professional storm chasers, and the rise of social media, warning forecasters now have greater access to real-time information than ever before.
Nearly as important, spotters provide much-needed postevent verification; Brotzge and Erickson (2010) found a systematic increase in the numbers of weak tornadoes verified over densely populated counties when compared with rural counties. However, erroneous reports from the field can impede the warning process; Smith (1999) describes how poor tornado verification overinflates tornado POD and overestimates the FAR. Brotzge et al. (2011) found very high FAR in high-population-density counties and very low FAR in sparsely populated counties, perhaps indicative of lower warning rates across rural areas because of the prevalence (or lack) of field reports available and a subsequent decrease in forecaster confidence for warning in those areas; see “Detection challenges” for more information.
DETECTION CHALLENGES
The most common reasons for operational warning forecasters for not detecting (and thereby not warning) tornadoes prior to touchdown often can be traced to having either too little information available—because of inadequacies in existing technology (e.g., LaDue et al. 2010 ), limited spotter networks, and incomplete conceptual models—or too much information, that is, data overload.
As the primary tool used for detecting tornadoes, weather radar is critical for seeing low-level to midlevel rotation prior to tornadogenesis. In areas with limited low-level radar coverage, tornado detection (and prediction) is severely hampered. In a root cause analysis study of 146 unwarned tornadoes between 2004 and 2009, “radar sampling,” “no radar signature,” and ”radar use” were listed as 3 of the top 10 reasons for failure to warn and were cited in over two-thirds of all missed events ( Quoetone et al. 2009 ). Sampling issues were cited in 19 of 31 false alarm events evaluated. Brotzge and Erickson (2010) found a mean 20% increase in the number of tornadoes not warned with increasing distance from radar, once sorted by population density.
Solutions to improving radar coverage include the use of lower-elevation scans, deployment of gap filling and rapid-scan radars, and an optimization of the radar network configuration. The WSR-88Ds' lowest scanning angle is 0.5° elevation, as limited by Federal Communications Commission (FCC) regulations. At some mountain sites across the western United States, the WSR-88D radars are located on mountain tops, limiting the views of critical valley areas. One solution now being implemented at a few locations is the use of zero and/or negative elevation angles ( R. Brown et al. 2002 , 2007 ; Wood et al. 2003 ). A second, long-term solution to improve radar coverage is to simply add more radars to the network. However, because of the high cost associated with deploying and operating large antenna, S-band (WSR-88D type) radars, a more cost-effective solution may be to deploy limited numbers of “gap filling” (X or C band) radars to fill in coverage gaps between WSR-88Ds ( McLaughlin et al. 2009 ). Brotzge et al. (2010) and Mahale et al. (2012) demonstrated the value of gap-filling radars for improving detection of tornado radar signatures. A third option for improving radar coverage is to sample more frequently. Replacement of the WSR-88Ds with rapid-scan, phase-darray radar (PAR) technology (e.g., Zrnić et al. 2007 ) could provide 1-min volume scans (or faster single elevation scans), an improvement over the current 4–6-min volume scans provided by the WSR-88Ds. In ongoing evaluations of the impact of PAR data on tornado warnings, Heinselman et al. (2012 , 2013) found that the use of faster scans has the potential to extend tornado warning lead times, reduce false alarms, and increase forecaster confidence. Finally, a more rigorous, optimal radar network configuration could improve overall low-level coverage. NEXRAD radars were originally deployed to operate as single autonomous systems; however, merged, multiradar data have proven more effective for extracting severe weather information ( Lakshmanan et al. 2006 ). Geometric, statistical, and genetic algorithm techniques have been developed to optimize the low-level coverage and maximize multi-Doppler overlap ( Ray and Sangren 1983 ; de Elía and Zawadzki 2001 ; Minciardi et al. 2003 ; Junyent and Chandrasekar 2009 ; Kurdzo and Palmer 2012 ). Nevertheless, the addition and/or replacement of radars will require a significant financial public investment.
Storm spotters provide an equally critical role to the warning forecaster. In the root cause analysis study, a lack of, conflicting or erroneous spotter reports were cited as having contributed to warning failure in nearly two-thirds of all missed events, and a lack of reports contributed to 15 of 31 false alarms ( Quoetone et al. 2009 ). Sustained education and coordination of spotter groups requires dedicated NWS resources. Fortunately, as described previously, access to real-time information and video from the field is becoming easier, with the proliferation of new video and wireless technologies (e.g., Dixon et al. 2012 ).
A basic understanding of tornado dynamics is still key to good forecasting and detection. In the Quoetone et al. (2009) root cause analysis study, “not anticipated,” “conceptual model failure,” and “environment” were listed among the top six reasons for warning misses. Poor radar, environmental conceptual models, and environment were listed as three of the top four reasons cited for issuing tornado false alarms. “Fits radar conceptual model” was cited in 30 of the 31 false alarm events studied. Continued improvement in the conceptual models requires sustained advances in basic research. Field programs such as the Verification of the Origins of Rotation in Tornadoes Experiment (VORTEX; Rasmussen et al. 1994 ) and the second VORTEX project (VORTEX2; Wurman et al. 2012 ) provide valuable observational data from which to study and improve understanding. Continued meteorological training and education are essential for moving research to operations.
Finally, with the plethora of new sensors and model output now available to the warning forecaster, many are now experiencing data overload, which is hampering warning operations. “Workload” was cited in one-third of all missed warnings, with “distractions” cited in one-quarter of all missed warnings ( Quoetone et al. 2009 ). One solution to this is the use of integrated, “fused” and/or assimilated sensor products (e.g., Wang et al. 2008 ). A second, complementary solution is the advent of multisensor and three-dimensional visualization (e.g., Gibson Ridge Software, LLC).
Once the formation of a tornado is considered likely or is reported already in progress, the NWS issues a tornado warning , the official NWS product used to warn the public of a tornado. The first tornado warning was issued on 25 March 1948 by U.S. Air Force officers E. Fawbush and R. Miller at Tinker Air Force Base in Oklahoma City, Oklahoma, and was remarkably successful ( Miller and Crisp 1999b ; Maddox and Crisp 1999 ). In fact, this first warning was so successful that it provided the scientific underpinning for establishment of the Air Weather Service Severe Weather Warning Center (SWWC), the first national severe weather warning program. During its first year of operation in 1951, the SWWC issued 156 (multicounty) tornado warnings, of which 102 (65%) were verified ( Miller and Crisp 1999a ). Since that time, tornado warnings, now issued by the local NWS Weather Forecast Offices (WFOs), have continued to improve as measured by the total percentage of tornadoes warned.
The final decision by the operational forecaster on whether to issue a warning is based upon a number of complex, sometimes competing factors. These factors may include environmental data, access to real-time weather and storm spotter information, forecaster experience, knowledge, distance of event from the nearest radar, population density, population vulnerability, tornado climatology, event anticipation, SPC guidance, and/or storm history. The interpretation of such data may be impacted by such things as personal fatigue, office staffing, and interoffice relationships. Andra et al. (2002) provides an excellent case study of warning decision making during the 3 May 1999 tornado outbreak in central Oklahoma.
Despite the difficulty of each decision, the warning forecaster strives to warn on every tornado, with as much lead time as possible, while minimizing the number of false alarm warnings. Having every tornado warned is essential for public safety; the public is much more likely to take shelter once they have received an official warning ( Balluz et al. 2000 ). However, there is an incentive to keep the warning area size small; the use of smaller warning polygons is estimated to save over $1.9 billion annually in reduced interruption and unnecessary sheltering ( Sutter and Erickson 2010 ). County-based tornado warnings were replaced with storm-based warning polygons in 2007.
As of 2011, the national tornado POD was 0.75, with a mean lead time of 14.6 min, and a FAR of 0.74 ( NOAA 2011b ). A review of the long-term trends in these statistics reveals that the POD and mean lead time have increased dramatically since the installation of the WSR-88D network and NWS modernization program ( Friday 1994 ), with a POD of 0.48 and a mean lead time of 7.6 min in 1994. However, nearly all of this increase in lead time was a direct result of greater numbers of tornadoes being warned ( Erickson and Brooks 2006 ); all tornadoes not warned were assigned a lead time of zero, and then included in the calculation of the mean lead time. Using data between 1986 and 2004, Erickson and Brooks recalculated tornado lead time without the missed tornadoes included and found a rather steady lead time of around 18.5 minutes. While greater numbers of tornadoes are being warned in advance (possibly because of improved radar technology, conceptual models, and training), lead time on warned tornadoes has not increased, and the FAR has remained steady at around 0.75 as well; see “Warning decision challenges” for more information.
WARNING DECISION CHALLENGES
A significant challenge to the forecaster is reducing the FAR while keeping the POD steady or improving ( Brooks 2004 ). Yet the value provided by the statistical measures (POD, FAR) is ambiguous. For example, POD is dependent largely upon the level of verification. FAR fails to account for close calls ( Barnes et al. 2007 ) and varies with parameters such as tornado order, climatology, and distance from radar ( Brotzge et al. 2011 ). While the POD, FAR, and warning lead time are frequently cited indices for measuring our ability to warn, additional improvement in these numbers may not translate necessarily into a reduction in tornado casualty rates. All of the deaths from the 27 April 2011 tornado outbreak occurred from tornadoes within active tornado watches and were preceded by tornado warnings ( NOAA 2011a ). Reasons for the high number of casualties from the April event include the rapid speed and severity of the event, the high population density of the areas hit, and delays in seeking shelter. Simmons and Sutter (2008) found warning lead times >15 min had little additional impact on fatality rates.
The impact of false alarms on public response is unknown. Early research found that false alarms may unexpectedly increase the likelihood of future response ( Janis 1962 ). More intuitively, Simmons and Sutter (2009 , p. 38) found that “a one-standard-deviation increase in the false-alarm ratio increases expected fatalities by between 12% and 29% and increases expected injuries by between 14% and 32%.” Other studies (mostly qualitative case studies) remain divided on the role of false alarms in the response process ( Breznitz 1984 ; Atwood and Major 1998 , Dow and Cutter 1998 ; Barnes et al. 2007 ).
A second emergent challenge for the warning forecaster is how to best blend information from automated algorithms, nowcasting, and NWP model output with conceptual models and human experience ( Stuart et al. 2006 ). At least in the short term, such output have limitations (e.g., Andra et al. 2002 ), and their use may be limited best as a check or calibration against the conceptual model.
Warning the public remains difficult in large part because the “public” is a largely diverse population with tremendous variation in education, physical abilities, family support, and situational awareness. To overcome these challenges, a variety of communication alert systems are used. Warnings may reach the public directly from the NWS through the National Oceanic and Atmospheric Administration (NOAA) Weather Radio (NWR) and the Internet, or indirectly through media, emergency management, and private sector weather providers. Widely adopted following the April 1974 tornado outbreak ( Coleman et al. 2011 ), NWR allows for an in-home method for waking a person from sleep in case of an emergency through its alert tone. Today, over 1,000 NWR transmitters offer 98% national coverage ( Zubrick 2010 ). The NWS also provides direct information to the general public via the Internet with some WFOs now experimenting with social media to distribute warning information.
The public most commonly receives tornado warnings from local media through television and radio (e.g., Hammer and Schmidlin 2002 ). Media utilize a host of methods to catch each viewer's attention and to convey the necessary information, including the use of “cutins,” “crawlers,” mobile phone apps, Facebook, and Twitter ( Coleman et al. 2011 ). Storm video and radar imagery provide greater spatial and temporal information regarding storm size, severity, storm motion, and geographic impact. Video media also more easily convey nonverbal cues from the television (TV) meteorologist. Indeed, research demonstrates that local populations often develop profound psychological commitments to specific weather stations or forecasters ( Sherman-Morris 2006 ). Television broadcasts are often simulcast over the radio but without the benefit of the images.
Emergency managers also play a critical role in disseminating weather information to the local community. As part of their responsibilities, EMs operate local warning systems, such as local outdoor warning sirens or reverse 911 systems, and coordinate disaster response and recovery efforts. An instant messaging service called NWSChat was created to facilitate direct communication between the NWS and EMs and to better support EM services. However, there are few consistent criteria applied across jurisdictions for warning dissemination. A number of meteorological (e.g., presence of a wall cloud) and nonweather-related (e.g., public backlash for issuing false alarms) factors influence the judgment of EMs on whether to activate warning systems ( Sorensen and Mileti 1987 ; Stewart and Lusk 1994 ; Donner 2008 ); see “Warning dissemination challenges” for more information.
WARNING DISSEMINATION CHALLENGES
A significant challenge in improving warning dissemination is to integrate new technologies in such a manner that those less able to afford such tools can still be warned. The Commercial Mobile Alert System (CMAS), Wireless Emergency Alerts (WEA), and Interactive NWS (iNWS) were recently created to disseminate warnings to mobile devices. However, many are ill equipped to receive text messaging, and so older warning systems, such as outdoor warning sirens, must still play a critical role within an integrated warning system, even as new, more informative services are made available.
The limitations of dissemination tools must be clearly recognized when building a public warning dissemination system. For example, mobile phone applications fail if and when cell phone towers and communications are disabled, a frequent problem in storm-ravaged areas. Similarly, outdoor warning sirens fail when power is lost to those sirens, such as occurred in some areas during the Alabama tornadoes of 27 April 2011. The use of outdoor sirens also varies significantly among jurisdictions, with some districts using them to warn on all severe thunderstorm (and sometimes nonweather)-related warnings, while other municipalities limit the use of sirens to tornado warnings only. Furthermore, many areas simply do not have sirens available, nor would it be cost effective to install sirens in many regions of the country. However, the consequences of not having sirens can be deadly; two people died in the 2011 Alabama tornadoes when early morning storms knocked out power to their trailer, and because they lived out of range of the nearest sirens, had no warning before they were hit ( Ammons 2011 ). Some jurisdictions have replaced all outdoor sirens with calling systems such as reverse 911. However, these systems have been known to take tens of minutes to call all those in the tornado path, with no guarantee that those called would be alerted prior to tornado impact. A battery-operated NWR provides an immediate and direct warning method, but NWR ownership is low with limited surveys showing ownership of ~10%–33% ( Manning 2007 ; Kupec 2008 ). NWR often is cited as the least-used method for obtaining warnings; only 3% of 1,650 persons surveyed just after the 3 May 1999 tornado in Moore, Oklahoma, indicated they had received their warning from NWR (S. Brown et al. 2002 ). While each system has certain limitations, an integrated and redundant dissemination system is more robust. In a survey following the 3 May 1999 Moore, Oklahoma, tornado, 55% of residents interviewed received the warning from more than one source ( Hammer and Schmidlin 2002 ).
Another challenge for the operational forecaster is how to effectively communicate scientific information to the general public. Instantaneous communication and the growth of meteorological support companies have had a significant impact on the warning process ( Golden and Adams 2000 ). As a result, institutions now communicate risk with unprecedented speed. Nevertheless, problems related to the expertise of institutions may affect the process of risk communication. For example, a recent experiment simulating a tornado outbreak tasked EMs with accessing and interpreting radar data ( Baumgart et al. 2008 ). Despite general competence, study participants experienced significant difficulties interpreting wind velocity data and, more importantly, synthesizing multiple forms of radar data to produce overall judgments, which affected the risk communication process.
Effective communication also entails that the public understands and makes effective use of warnings ( Lazo 2012 ). The risk communication process is most effective when those at risk hold a “perceived shared experience” with those already victimized ( Aldoorya et al. 2010 ). When those warned could relate to victims (e.g., similar gender or race), threat acknowledgment and information seeking increased. Thus, risk communication may be taken more seriously if nearby communities are affected. How warnings are communicated also may shape risk communication. Numerical representations of risk often fail to persuade ( Lipkus and Hollands 1999 ). In an experiment on risk perception of flooding, images depicting flood damage reinforced perceived risks ( Keller et al. 2006 ). NOAA is now conducting an impact-based warning experiment ( Maximuk and Hudson 2012 ) to evaluate ways in which to improve NWS communication to motivate improved public response.
PUBLIC RESPONSE.
Warning dissemination sets into motion a process of public response, a complex and multidimensional activity. While research on risk and warning response has been conducted since the 1950s, it was not until the 1990s that scholars began to systematize findings into a general model. Mileti and Sorensen (1990) and Lindell and Perry (1992) shared the common conclusion that warning response was not a single act, but a set of stages through which the public progressed in responding to disseminated warnings. Before taking action, the public must receive, understand, believe, confirm, and personalize warnings.
Community members receive warning information through formal and informal channels. Formal communication includes NWS, media, emergency management, and reverse 911, or any official warning system. Informal communication includes family, friends, and coworkers. Each form of communication channels warning information to the public, but each does so in dramatically different ways. Formal communication tends to reach members of upper- and middle-class populations, while informal communication often better serves the poor, ethnic minorities, and recent migrants. For instance, warnings issued during the 1987 Saragosa, Texas, tornadoes failed to reach local Hispanic populations ( Aguirre 1988 ; Ahlborn and Franc 2012 ). Latinos prefer friends and family as sources of warning information ( Peguero 2006 ) and receive tornado warning information from informal networks ( Donner 2007 ). Poorer populations also were less likely to receive formal warnings ( Schmidlin and King 1997 ).
Social networks may play a key role in reception. For instance, Nagarajan et al. (2012) documented the importance of warning dissemination among neighbors in a series of computer simulations. Frequent interaction of family members ( Lardry and Rogers 1982 ), strong community or network involvement ( Turner et al. 1979 ; Sorensen and Gersmehl 1980 ; Perry and Greene 1983 ; Rogers and Nehnevajsa 1987 ; Rogers and Sorensen 1991 ), regular association with a subculture or voluntary association ( Perry et al. 1981 ), and more frequent community interaction ( Scanlon and Frizzell 1979 ) improved the likelihood of message reception among individuals within the community.
How recipients understand and make sense of warning information is deeply connected to human psychology and past experience. With the exception of Quarantelli (1980) , research overwhelmingly demonstrates that long-term residents generally tend to hold a better understanding of warning information ( Haas et al. 1977 ; Foster 1980 ; Perry and Greene 1983 ; Perry and Lindell 1986 ; Blanchard-Boehm 1998 ). Psychologically, the public is more likely to understand warning information if conveyed along with local information and maps ( Berry 1999 ). Multiple warning sources increase chances of comprehension ( Mileti and Darlington 1995 ), while at the same time excessive information within a single message may lead to higher rates of misunderstanding ( DiGiovanni et al. 2002 ). Probability information attached to tornado warnings (e.g., the tornado has a 30% chance of occurring), for instance, may confuse rather than clarify risks for the public ( Morss et al. 2010 ).
One concern is whether individuals understand the difference between warnings and watches. In a study of Austin, Texas, residents, Schultz et al. (2010) found that 90% of the sample could adequately distinguish between watches and warnings. Other studies found similar rates of understanding ( Balluz et al. 2000 ; Biddle and Legates 1999 ), while others encountered more modest results ( Mitchem 2003 ). Still other research suggests much lower rates of comprehension. In a broad survey of 769 residents across Texas, Oklahoma, and California, only 58% of all participants correctly understood the difference between a watch and a warning, though the percentage improved among residents in Oklahoma and Texas and among older and more educated survey participants ( Powell and O'Hair 2008 ).
Social scientists have identified a number of social and cultural factors that account for variation in warning comprehension between individuals. Education is consistently associated with greater understanding ( Turner et al. 1979 ), and those with a greater familiarity with science and scientific concepts generally hold a stronger understanding of warnings ( Blanchard-Boehm 1998 ). Age, too, shows a direct correlation with understanding ( Turner et al. 1979 ; Blanchard-Boehm 1998 ).
After understanding a warning message, the recipient evaluates the credibility of the message. Will there really be a tornado or is the warning a false alarm? In other words, should the message be taken seriously? Rarely, however, at this stage do recipients arrive at a concrete conclusion about whether a tornado will or will not occur. On the contrary, recipients crudely evaluate the probability of severe weather. The psychological qualities, past experiences, and unique demographic characteristics of the individual play a significant role in shaping these judgments of likelihood.
Those closer to a hazard are more likely to believe a warning ( Diggory 1956 ; Sorensen 1982 ), which may be because of the greater likelihood of experiencing environmental cues ( Drabek 1969 ; Quarantelli 1980 ; Sorensen 1982 ; Tierney 1987 ; Mileti and Fitzpatrick 1993 ; Hammer and Schmidlin 2002 ). Additional psychological processes also may play a significant role in the process of believing warnings. There are mixed findings regarding whether certain sources are more or less believable. Some research shows the public places greater faith in “official sources” (e.g., NWS warnings; Li 1991 ; Drabek 1994 ), whereas other studies routinely demonstrate “unofficial sources” (e.g., family) to hold greater credibility among the communities ( Sorensen 1982 ; Perry 1983 ; Li 1991 ). It may be that the particular source may play a lesser role in credibility when compared to source familiarity. Warning sources to which individuals are personally or emotionally attached (e.g., a favorite weather forecaster) or with which they are more familiar may appear more credible ( Mileti and Fitzpatrick 1993 ).
Demographic factors have some influence as well. Women appear more likely to believe warnings ( Drabek 1969 ; Farley et al. 1993 ; Sherman-Morris 2010 ). Why this is the case may be explained through socialization, as well as the fact that women tend to be caregivers ( Perry 1983 ). Additionally, the higher one's socioeconomic class, the more likely one is to believe a warning ( Sorensen 1982 ; Perry 1987 ). Finally, a society's culture may also play a role in warning response. Finding the Japanese more likely than U.S. residents to respond to volcano warnings, Perry and Hirose (1991 , p. 112) explain that Japanese live within a “collectivist culture in which citizens have higher expectations that authorities will provide care in the event of disasters or other disruptions in social life.” Perry and Hirose suggest that the Japanese population has greater trust in government, and thus greater response rates, than Western societies, and that response to warnings among the Japanese might reflect the broader cultural rules of obedience and authority common in Asian societies.
A common feature of the warning process ( Mileti 1999 ), confirmation serves to clarify and specify warning information, but at the cost of delaying sheltering. Confirmation has been found to take place among neighbors, rather than through formal channels ( Kirschenbaum 1992 ), with information from media sources more likely the subject of confirmation ( Frazier 1979 ). Confirmation may also be something as simple as visual confirmation of the storm. Whether beneficial or detrimental, confirmation remains a certain feature of the warning process.
Risk personalization deals with whether community members believe severe weather will affect them personally. In other words, one can believe that a threat exists somewhere , but the threat is not immediate and therefore action is unnecessary. For example, residents may decide that the mountains or rivers surrounding their community protect them from tornadoes, even if they believe local reports that storms may produce tornadoes ( Donner et al. 2012 ).
The psychological elements of risk personalization are well understood. Warning consistency yields greater personalization of risk ( McDavid and Marai 1968 ; Lindell and Perry 1983 ). Warning specificity ( Perry et al. 1981 ) and sender credibility ( Perry 1979 ; Rogers and Nehnevajsa 1987 ) contribute to personalization. Geographical proximity to a threat appears to be the most important in the literature ( Flynn 1979 ; Perry and Lindell 1986 ; Rogers and Nehnevajsa 1987 ). With some notable dissent ( Mileti and Darlington 1995 ), most research agrees that past hazards experience leads to a greater likelihood of personalization ( Perry 1979 ; Hansson et al. 1982 ; Saarinen et al. 1984 ; Rogers and Nehnevajsa 1987 ).
Demographics also play a role. As with belief, women are more likely to personalize a threat ( Flynn 1979 ; Hodge et al. 1981 ). Socioeconomic status also may play a role in risk personalization ( Flynn 1979 ; Mileti et al. 1981 ).
Believing that one is personally at risk sets off a process of determining whether one must and is able to do something to protect oneself. Little research has been conducted in this area of the model. This stage is unique from resource availability, in that resources may be available but the potential victim either does not know about them or does not think them useful for protection.
Protection from severe weather often takes the form of sheltering. Sheltering may be broadly defined as either “in home” or “public.” With in-home sheltering, refuge is typically sought in hallways, closets, underground basements, or, ideally, personal shelters. Those under warning may also choose to seek public shelters, which are typically set up and maintained by local government. Public shelters may be stand-alone shelters, in that their only use is as a shelter, or schools, town halls, or other municipal structures may become “shelters” during storms. Education, possibly through increased income, is most consistently associated with the availability of resources such as shelters ( Edwards 1993 ; Balluz et al. 2000 ); see “Public response challenges” for more information.
PUBLIC RESPONSE CHALLENGES
Although the determinants of shelter seeking are well documented in the literature, little is known about the sheltering process itself. Personal shelters are ideal, in that sheltering is immediate; traveling to a public shelter may be dangerous, especially in the context of tornadoes that are rapid and violent on onset. For those in mobile homes or similar vulnerable structures without shelters, evacuation may be the only option; mobile homes comprised 7.6% of U.S. housing stock in 2000, but 43.2% of all tornado fatalities between 1985 and 2007 occurred in mobile homes ( Sutter and Simmons 2010 ). In addition to distance, other more “human” factors may shape the use of shelters. Cola (1996) found that people were less likely to use shelters thought uncomfortable. Pet owners also may be less likely to seek shelter ( Heath 1999 ; Pfister 2002 ). More research is needed to understand shelter use and its relationship to lead time and social factors. Additional work needs to explore the associated needs, optimal locations, and operation of public tornado shelters.
There is also the real inability by some to take shelter because of disability. In the Joplin, Missouri, tornado, three mentally handicapped men died when their home was hit. Also in Joplin, 12 residents and a nursing assistant died at the Greenbriar nursing home, and another 8 patients died when St. John's Regional Medical Center was hit. Both facilities had been warned and had begun taking storm precautions, but neither had enough lead time to evacuate. In Shoal Creek, Alabama, seven people were killed when an assisted living facility was hit. Additional research is needed to explore the lead time requirements for those who must evacuate (e.g., from trailer homes) or need help sheltering (e.g., those with special needs). Indeed, the public at large requires a continuum of lead times, where for some a warning lead time of well over 30 min could be essential, whereas for others, a large lead time could lead to apathy and greater danger.
All other things being equal, as the U.S. population density increases, tornado fatalities may be expected to increase, calling for a review of the prediction, detection, and communication processes through which tornadoes are warned. Urban populations continue to rise in hazard prone regions, thereby placing greater numbers of people at risk ( Brooks and Doswell 2001 ; Ashley 2007 ). In addition, the overall population is aging, with increasing numbers living alone ( Gusmano and Rodwin 2006 ). Greater diversity among the population introduces additional challenges, such as warning dissemination to non-English-speaking populations ( Donner and Rodríguez 2008 ). As described herein, a number of challenges limit the effectiveness of the current warning system ( Table 1 ). Based upon the preceding literature review and these associated challenges, the warning process can be fundamentally improved with a greater emphasis and understanding of acceptable risk , preparation , and personalization .
List of tornado warning system challenges.
A fundamental question society must ask is, “How much risk are we willing to tolerate?” The answer to this dilemma will set the limit on how much money should be expended toward further research and warning infrastructure. In other words, the public must define its acceptable risks, and its willingness to provide additional resources or reduce existing services or quality to match those risks ( Stallings 1990 ). The public's level of acceptable risk likely varies across the country as a function of the nature and extent of the risk. This variability calls for an emphasis on local-to-regional decision making, such that any top-down, one-size-fits-all strategy will likely be less than optimal. A dense observing spotter and warning dissemination network in the plains may vary in function and form from one in the Southeast, whereas neither system may be cost effective in the West or New England.
A second essential subject often overlooked in discussions of the tornado warning process is preparation, both at the organizational and personal levels. Preparation at the organizational level may include the development of public policy regarding the use and availability of public shelters and warning systems, the availability of multilingual warnings, requirements or guidelines for shelters in mobile home parks, building codes, and sheltering procedures. Private preparations may include developing a family disaster plan, copying and storing critical insurance papers and photos in safety deposit boxes, or purchasing a safe room or shelter. Proper preparation at the organizational level can often facilitate the speed and ease of personal decision making during a moment of crisis.
Preparation should focus on maximizing personal safety, minimizing economic loss, and easing recovery efforts. While this article has focused on public safety, total damage estimates from tornadoes between 1950 and 2011 range from $300 billion to $450 billion (U.S. dollars; Simmons et al. 2013 ). A greater focus on personal mitigation could reduce tornado damage. Sutter et al. (2009) found that low-cost home mitigation could reduce tornado damage by as much as 30%.
Finally, the one common ingredient to a successful end-to-end tornado warning program is the personalization of the warning; to be successful, warnings must evoke a sense of specific and immediate risk. Even days prior to an event, the efforts of the SPC and others are spent narrowing the area of a potential threat; local WFOs narrow the threat further in time and space, issuing warnings over specific regions in time. The most effective warnings are those that communicate clearly to individuals the specific information they need to know with enough time to react. The goals of ensemble NWP, warn on forecast, phased array and gap-fill radars, and storm-based warnings are to provide more detailed data on when and where tornadoes will strike. Many new and innovative warning dissemination tools, many developed and sold by the private sector, convey this detailed information to individuals, through the use of local media, outdoor warning sirens, NOAA Weather Radio, the Internet, smart phones, and pagers. Similarly, preparation for tornadoes needs to be personalized, and specific mitigation information provided at a household level could see potential dividends in reducing home damage and personal injury.
Social and cultural factors may inhibit personalization of warnings. Long lead times and high false alarm rates tend to depersonalize risk. A continuing program of research and education remains key to systematically improving public response to warnings.
A highly integrated and efficient tornado warning system does not necessarily ensure that no fatalities will ever occur, but it does set a priori standards of warning capability as a function of the community-defined level of acceptable risk, resources, and will. The effectiveness of the best tornado warning system is dependent largely upon the comprehensiveness and manner of preparedness at the organizational and personal levels. This review has demonstrated the value of research and investment at all stages of the warning process for improving the personalization of the warning. In an era of austerity, additional investments will need to be strategically focused to further prepare and personalize the tornado threat.
ACKNOWLEDGMENTS
This work is supported by the Engineering Research Centers Program of the National Science Foundation under NSF Award 0313747. The authors thank three anonymous reviewers for their insightful comments and suggestions for the improvement of this manuscript. Any opinions, findings, conclusions, or recommendations expressed in this material are those of the authors and do not necessarily reflect those of the National Science Foundation.
AMS Publications

Get Involved with AMS
Affiliate sites.
Email : [email protected]
Phone : 617-227-2425
Fax : 617-742-8718
Headquarters:
45 Beacon Street
Boston, MA 02108-3693
1200 New York Ave NW
Washington, DC 200005-3928
- Privacy Policy & Disclaimer
- Get Adobe Acrobat Reader
- © 2024 American Meteorological Society
- [185.80.151.41]
- 185.80.151.41
Character limit 500 /500
NSSL NOAA National Severe Storms Laboratory
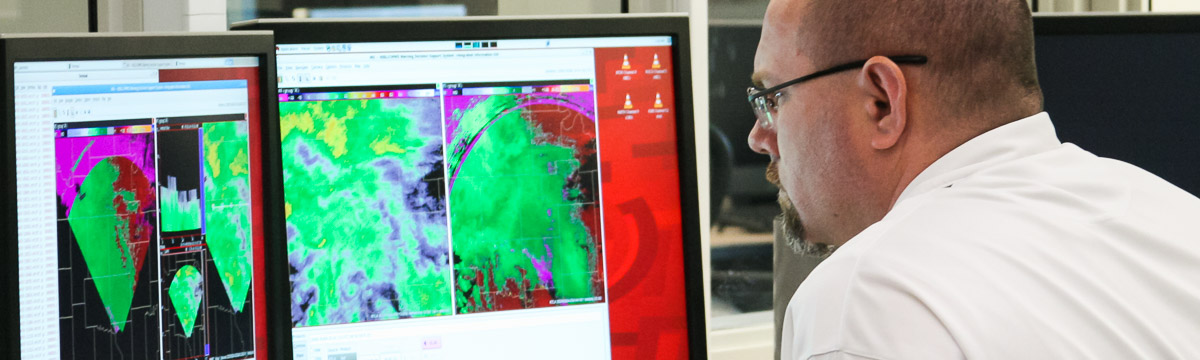
Research at NSSL
Severe weather has touched every state in the United States. Hurricanes, tornadoes, blizzards, wildfires, floods and droughts are very real threats to our property and our lives. NSSL researchers work to observe, understand and predict severe weather in ways that will help our partners save lives and reduce property damage.
Thunderstorms
At NSSL we study all types of thunderstorms including supercell thunderstorms, mesoscale convective systems, quasi-linear convective systems and bow-echoes. We also study their environment and their life-cycle.
Read more →
Much about tornadoes remains a mystery. They are rare, unpredictable and deadly. The U.S. has more tornadoes than anywhere else in the world. NSSL scientists study the lifecycles of tornadoes, why some supercells produce tornadoes and others do not, and what exactly causes a tornado to form. We also look for ways to improve tornado warning accuracy and lead-time.
Except for heat related fatalities, more deaths occur from flooding than any other hazard ( NWS Jetstream ). NSSL flood research focuses on improving ways to monitor water levels and precipitation amounts in ways that will improve flood and flash flood forecasts and warnings.
Lightning not only injures and kills people, it also ignites forest and brush fires. NSSL scientists find unique ways to measure and study lightning in the field. We also create computer simulations of lightning, and look for ways to use lightning data in forecasts of severe weather.
Hail can cause billions of dollars of damage to structures, crops and livestock. NSSL hail research focuses on improving detection and warning of hail to give people time to protect their property and seek shelter.
Damaging Winds
Straight-line winds are responsible for most of the damage from thunderstorms. These winds can cause as much destruction as a strong tornado. NSSL works to better understand the thunderstorms that produce damaging winds, so the NWS can make better predictions and warnings for them.
Winter Weather
Forecasting winter weather accurately is difficult because a degree or two of temperature change can mean the difference between snow or freezing rain. NSSL research includes looking for ways to make forecasting winter precipitation easier.
Societal Impacts
NSSL researchers work to understand and provide the weather information society needs. NSSL social science research directly involves emergency managers, broadcast meteorologists, and operational forecasters, in addition to the United States public, to assure innovations in weather research are holistically integrated into the weather communication system.

COMMENTS
Introduction. Recent devastating tornado outbreaks in the southeast United States (SEUS), for example, the April 2011 outbreak which produced 25 killer tornadoes [], have heightened the need to better understand physical and social vulnerability to tornadoes in the region.After the April 2011 outbreak, researchers found that residents based their decisions during the event, in part, on their ...
NSSL Research: Tornadoes. Much about tornadoes remains a mystery. They are rare, deadly, and difficult to predict, and they can deal out millions or even billions of dollars in property damage per year. The U.S. typically has more tornadoes than anywhere else in the world, though they can occur almost anywhere.
In the United States, tornado outbreaks have substantial effects on human lives and property. Tornado outbreaks are sequences of six or more tornadoes that are rated F1 and greater on the Fujita scale or rated EF1 and greater on the Enhanced Fujita scale and that occur in close succession (1, 2).About 79% of tornado fatalities during the period 1972 to 2010 occurred in outbreaks (), and 35 ...
Modern logging of tornado reports in the U.S. has begun in the 1950s, and the reports grew even more in the mid-1990s, mainly due to the increase in the detection of EF0-EF1 category tornadoes ...
A highly-idealized and simple tornado model is examined in Markowski et al. (2014)), as illustrated in Fig. 2 (Markowski, 2019), which highlights the role of a downdraft in transporting vorticity to very near the ground.Other studies indicate that vorticity in parcels that comprise a tornado can be created via baroclinic generation above the near-ground layer, and crosswise-streamwise exchange ...
The Tallulah-Yazoo City-Durant tornado (Louisiana and Mississippi) of 24 April 2010 that killed 10 and injured 146 had an estimated power of 66,200 GW. Annual statistics of tornado power show clear upward trends with the median, quartiles, and 90th percentile all on the rise over the period 1994-2016 (Figure 1).
Of greatest interest here is the potential for shifts in the spatial location of tornado frequency. Research has identified evidence of a "Dixie Alley," which represents an eastward extension ...
This study documents the actual distribution of supercell-tornado wind intensities and sizes, revealing that most are much stronger than damage surveys indicate, with >20% of tornadoes poten-tially capable of causing catastrophic EF-4/EF-5 damage. Addi-tionally, supercell tornadoes are shown to be much wider than damage surveys indicate.
The trend in NT tornado deaths per 1 million persons is consis-tent since 1970, ranging from 0.1 to 0.2 deaths per 1 million per-sons (Fig. 3a). Since 1880, the percentage of all fatalities that occurred during DT hours declined by 20% while the proportion of NT deaths nearly doubled.
As one of the most severe and high-impact weather phenomena, tornadoes and their long-term frequency trends have received lots of attention from both the scientific community and the public. Here, we show that over the last six decades (1954-2018), U.S. (E)F1 tornadoes have a statistically significant upward trend but (E)F2-E)F4 tornadoes have statistically significant downward trends ...
Abstract Tornadoes in the southeastern United States continue to cause substantial injury, death, and destruction. The present study seeks to 1) understand inadequate warning access, less understanding, and/or less likelihood of responding to tornado warnings; 2) examine public attitudes about NWS communications; and 3) explore the perceptions of NWS personnel regarding public response to ...
In the Tornado Alley, there has been a 34-69% increase in the rate of annual tornadoes since the 1990s. ... and hence motivated us to embark on this research of classifying the climatological trends across 28 major tornado-impacted states in the U.S. In general, this is a high-dimensional problem that offers interesting opportunities since ...
Previous studies have identified statistical linkages between tornado occurrences and supercell thunderstorm activity in the Great Plains region to atmospheric pressure anomalies near the Rocky Mountains (5, 6), dry lines (), the North American low-level jet (8, 9), the Madden-Julian Oscillation (10-12), the El Niño-Southern Oscillation (ENSO) (5, 13-16), and SSTA over the Gulf of ...
Foshan Tornado Research Center, and China Meteorological Administration Tornado Key Laboratory, Guangdong Meteorological Service, Foshan, Guangdong, China ... The present paper aims to investigate the causes of tornado outbreaks and the lack thereof within two analogous recurving TC scenarios that occur in East Asia. To achieve this goal ...
As a result of the nonmeteorological effects on the tornado database, much of the research has focused on developing covariates that relate environmental conditions to the occurrence of tornadoes and looking at distributions of those covariates in time and space, mimicking a process seen in operational weather forecasting (5-8).Unfortunately, the approach of using covariates has produced ...
In this paper we analyze the behavior of tornado time-series in the U.S. from the perspective of dynamical systems. A tornado is a violently rotating column of air extending from a cumulonimbus cloud down to the ground. Such phenomena reveal features that are well described by power law functions and unveil characteristics found in systems with long range memory effects.
The Newcastle/Moore and El Reno tornadoes of May 2013 are recent reminders of the destructive power of tornadoes. A direct estimate of a tornado's power is difficult and dangerous to get. An indirect estimate on a categorical scale is available from a post-storm survery of the damage. Wind speed bounds are attached to the scale, but the scale is not adequate for analyzing trends in tornado ...
In future research, if we search for papers written in multiple languages, we will be able to find data on tornadoes outside the United States and their aftermath. This attempt will add diversity and depth to tornado research, which is lacking in quantitative and qualitative compared to other natural hazards research. 5. Conclusions
Tornado Warning, Preparedness, and Impacts. Description: This special collection of Weather, Climate, and Society contains research on tornado hazards in three main areas. First, communication, perception, and response to tornado warnings is a major theme that is carried across through the role of, and design of, graphic communication, factors ...
With the unusually violent tornado season of 2011, there has been a renewed national interest, through such programs as NOAA's Weather Ready Nation initiative, to reevaluate and improve our tornado warning process. This literature review provides an interdisciplinary, end-to-end examination of the tornado warning process. Following the steps outlined by the Integrated Warning System, current ...
This study documents the actual distribution of supercell-tornado wind intensities and sizes, revealing that most are much stronger than damage surveys indicate, with >20% of tornadoes potentially capable of causing catastrophic EF-4/EF-5 damage. Additionally, supercell tornadoes are shown to be much wider than damage surveys indicate.
close proximity to tornadoes (Markowski, 2002). Further-more, trajectory analyses in a limited number of observed supercells indicate that at least some of the air entering the tornado passes through the RFD prior to entering the tornado (e.g., Brandes, 1978). Numerical simulation results also have emphasized the importance of the RFD and have
Research at NSSL. Severe weather has touched every state in the United States. Hurricanes, tornadoes, blizzards, wildfires, floods and droughts are very real threats to our property and our lives. NSSL researchers work to observe, understand and predict severe weather in ways that will help our partners save lives and reduce property damage.
More damaging than tornadoes, hail may finally get the scientific attention it deserves ... Such tools will bring hail research "into the 21st century," says Julian Brimelow, a meteorologist and executive director of Canada's Northern Hail Project, a research group involved in ICECHIP. But some technologies from the 1970s will remain ...