Thank you for visiting nature.com. You are using a browser version with limited support for CSS. To obtain the best experience, we recommend you use a more up to date browser (or turn off compatibility mode in Internet Explorer). In the meantime, to ensure continued support, we are displaying the site without styles and JavaScript.
- View all journals
- Explore content
- About the journal
- Publish with us
- Sign up for alerts
- Review Article
- Open access
- Published: 08 June 2021

Metacognition: ideas and insights from neuro- and educational sciences
- Damien S. Fleur ORCID: orcid.org/0000-0003-4836-5255 1 , 2 ,
- Bert Bredeweg ORCID: orcid.org/0000-0002-5281-2786 1 , 3 &
- Wouter van den Bos 2 , 4
npj Science of Learning volume 6 , Article number: 13 ( 2021 ) Cite this article
39k Accesses
52 Citations
11 Altmetric
Metrics details
- Human behaviour
- Interdisciplinary studies
Metacognition comprises both the ability to be aware of one’s cognitive processes (metacognitive knowledge) and to regulate them (metacognitive control). Research in educational sciences has amassed a large body of evidence on the importance of metacognition in learning and academic achievement. More recently, metacognition has been studied from experimental and cognitive neuroscience perspectives. This research has started to identify brain regions that encode metacognitive processes. However, the educational and neuroscience disciplines have largely developed separately with little exchange and communication. In this article, we review the literature on metacognition in educational and cognitive neuroscience and identify entry points for synthesis. We argue that to improve our understanding of metacognition, future research needs to (i) investigate the degree to which different protocols relate to the similar or different metacognitive constructs and processes, (ii) implement experiments to identify neural substrates necessary for metacognition based on protocols used in educational sciences, (iii) study the effects of training metacognitive knowledge in the brain, and (iv) perform developmental research in the metacognitive brain and compare it with the existing developmental literature from educational sciences regarding the domain-generality of metacognition.
Similar content being viewed by others
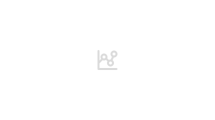
A methodological perspective on learning in the developing brain
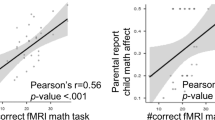
An fMRI study of error monitoring in Montessori and traditionally-schooled children
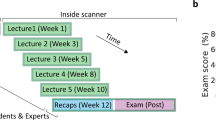
Neural alignment predicts learning outcomes in students taking an introduction to computer science course
Introduction.
Metacognition is defined as “thinking about thinking” or the ability to monitor and control one’s cognitive processes 1 and plays an important role in learning and education 2 , 3 , 4 . For instance, high performers tend to present better metacognitive abilities (especially control) than low performers in diverse educational activities 5 , 6 , 7 , 8 , 9 . Recently, there has been a lot of progress in studying the neural mechanisms of metacognition 10 , 11 , yet it is unclear at this point how these results may inform educational sciences or interventions. Given the potential benefits of metacognition, it is important to get a better understanding of how metacognition works and of how training can be useful.
The interest in bridging cognitive neuroscience and educational practices has increased in the past two decades, spanning a large number of studies grouped under the umbrella term of educational neuroscience 12 , 13 , 14 . With it, researchers have brought forward issues that are viewed as critical for the discipline to improve education. Recurring issues that may impede the relevance of neural insights for educational practices concern external validity 15 , 16 , theoretical discrepancies 17 and differences in terms of the domains of (meta)cognition operationalised (specific or general) 15 . This is important because, in recent years, brain research is starting to orient itself towards training metacognitive abilities that would translate into real-life benefits. However, direct links between metacognition in the brain and metacognition in domains such as education have still to be made. As for educational sciences, a large body of literature on metacognitive training is available, yet we still need clear insights about what works and why. While studies suggest that training metacognitive abilities results in higher academic achievement 18 , other interventions show mixed results 19 , 20 . Moreover, little is known about the long-term effects of, or transfer effects, of these interventions. A better understanding of the cognitive processes involved in metacognition and how they are expressed in the brain may provide insights in these regards.
Within cognitive neuroscience, there has been a long tradition of studying executive functions (EF), which are closely related to metacognitive processes 21 . Similar to metacognition, EF shows a positive relationship with learning at school. For instance, performance in laboratory tasks involving error monitoring, inhibition and working memory (i.e. processes that monitor and regulate cognition) are associated with academic achievement in pre-school children 22 . More recently, researchers have studied metacognition in terms of introspective judgements about performance in a task 10 . Although the neural correlates of such behaviour are being revealed 10 , 11 , little is known about how behaviour during such tasks relates to academic achievement.
Educational and cognitive neuroscientists study metacognition in different contexts using different methods. Indeed, while the latter investigate metacognition via behavioural task, the former mainly rely on introspective questionnaires. The extent to which these different operationalisations of metacognition match and reflect the same processes is unclear. As a result, the external validity of methodologies used in cognitive neuroscience is also unclear 16 . We argue that neurocognitive research on metacognition has a lot of potential to provide insights in mechanisms relevant in educational contexts, and that theoretical and methodological exchange between the two disciplines can benefit neuroscientific research in terms of ecological validity.
For these reasons, we investigate the literature through the lenses of external validity, theoretical discrepancies, domain generality and metacognitive training. Research on metacognition in cognitive neuroscience and educational sciences are reviewed separately. First, we investigate how metacognition is operationalised with respect to the common framework introduced by Nelson and Narens 23 (see Fig. 1 ). We then discuss the existing body of evidence regarding metacognitive training. Finally, we compare findings in both fields, highlight gaps and shortcomings, and propose avenues for research relying on crossovers of the two disciplines.
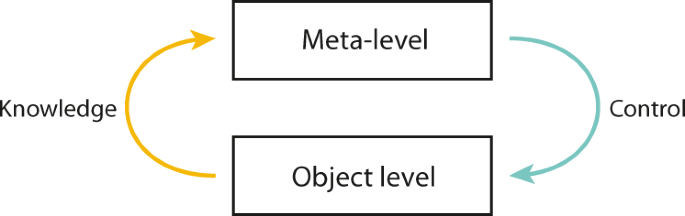
Meta-knowledge is characterised as the upward flow from object-level to meta-level. Meta-control is characterised as the downward flow from meta-level to object-level. Metacognition is therefore conceptualised as the bottom-up monitoring and top-down control of object-level processes. Adapted from Nelson and Narens’ cognitive psychology model of metacognition 23 .
In cognitive neuroscience, metacognition is divided into two main components 5 , 24 , which originate from the seminal works of Flavell on metamemory 25 , 26 . First, metacognitive knowledge (henceforth, meta-knowledge) is defined as the knowledge individuals have of their own cognitive processes and their ability to monitor and reflect on them. Second, metacognitive control (henceforth, meta-control) consists of someone’s self-regulatory mechanisms, such as planning and adapting behaviour based on outcomes 5 , 27 . Following Nelson and Narens’ definition 23 , meta-knowledge is characterised as the flow and processing of information from the object level to the meta-level, and meta-control as the flow from the meta-level to the object level 28 , 29 , 30 (Fig. 1 ). The object-level encompasses cognitive functions such as recognition and discrimination of objects, decision-making, semantic encoding, and spatial representation. On the meta-level, information originating from the object level is processed and top-down regulation on object-level functions is imposed 28 , 29 , 30 .
Educational researchers have mainly investigated metacognition through the lens of Self-Regulated Learning theory (SRL) 3 , 4 , which shares common conceptual roots with the theoretical framework used in cognitive neuroscience but varies from it in several ways 31 . First, SRL is constrained to learning activities, usually within educational settings. Second, metacognition is merely one of three components, with “motivation to learn” and “behavioural processes”, that enable individuals to learn in a self-directed manner 3 . In SRL, metacognition is defined as setting goals, planning, organising, self-monitoring and self-evaluating “at various points during the acquisition” 3 . The distinction between meta-knowledge and meta-control is not formally laid down although reference is often made to a “self-oriented feedback loop” describing the relationship between reflecting and regulating processes that resembles Nelson and Narens’ model (Fig. 1 ) 3 , 23 . In order to facilitate the comparison of operational definitions, we will refer to meta-knowledge in educational sciences when protocols operationalise self-awareness and knowledge of strategies, and to meta-control when they operationalise the selection and use of learning strategies and planning. For an in-depth discussion on metacognition and SRL, we refer to Dinsmore et al. 31 .
Metacognition in cognitive neuroscience
Operational definitions.
In cognitive neuroscience, research in metacognition is split into two tracks 32 . One track mainly studies meta-knowledge by investigating the neural basis of introspective judgements about one’s own cognition (i.e., metacognitive judgements), and meta-control with experiments involving cognitive offloading. In these experiments, subjects can perform actions such as set reminders, making notes and delegating tasks 33 , 34 , or report their desire for them 35 . Some research has investigated how metacognitive judgements can influence subsequent cognitive behaviour (i.e., a downward stream from the meta-level to the object level), but only one study so far has explored how this relationship is mapped in the brain 35 . In the other track, researchers investigate EF, also referred to as cognitive control 30 , 36 , which is closely related to metacognition. Note however that EF are often not framed in metacognitive terms in the literature 37 (but see ref. 30 ). For the sake of concision, we limit our review to operational definitions that have been used in neuroscientific studies.
Metacognitive judgements
Cognitive neuroscientists have been using paradigms in which subjects make judgements on how confident they are with regards to their learning of some given material 10 . These judgements are commonly referred to as metacognitive judgements , which can be viewed as a form of meta-knowledge (for reviews see Schwartz 38 and Nelson 39 ). Historically, researchers mostly resorted to paradigms known as Feelings of Knowing (FOK) 40 and Judgements of Learning (JOL) 41 . FOK reflect the belief of a subject to knowing the answer to a question or a problem and being able to recognise it from a list of alternatives, despite being unable to explicitly recall it 40 . Here, metacognitive judgement is thus made after retrieval attempt. In contrast, JOL are prospective judgements during learning of one’s ability to successfully recall an item on subsequent testing 41 .
More recently, cognitive neuroscientists have used paradigms in which subjects make retrospective metacognitive judgements on their performance in a two-alternative Forced Choice task (2-AFC) 42 . In 2-AFCs, subjects are asked to choose which of two presented options has the highest criterion value. Different domains can be involved, such as perception (e.g., visual or auditory) and memory. For example, subjects may be instructed to visually discriminate which one of two boxes contains more dots 43 , identify higher contrast Gabor patches 44 , or recognise novel words from words that were previously learned 45 (Fig. 2 ). The subjects engage in metacognitive judgements by rating how confident they are relative to their decision in the task. Based on their responses, one can evaluate a subject’s metacognitive sensitivity (the ability to discriminate one’s own correct and incorrect judgements), metacognitive bias (the overall level of confidence during a task), and metacognitive efficiency (the level of metacognitive sensitivity when controlling for task performance 46 ; Fig. 3 ). Note that sensitivity and bias are independent aspects of metacognition, meaning that two subjects may display the same levels of metacognitive sensitivity, but one may be biased towards high confidence while the other is biased towards low confidence. Because metacognitive sensitivity is affected by the difficulty of the task (one subject tends to display greater metacognitive sensitivity in easy tasks than difficult ones and different subjects may find a task more or less easy), metacognitive efficiency is an important measure as it allows researchers to compare metacognitive abilities between subjects and between domains. The most commonly used methods to assess metacognitive sensitivity during retrospective judgements are the receiver operating curve (ROC) and meta- d ′. 46 Both derive from signal detection theory (SDT) 47 which allows Type 1 sensitivity, or d’ ′ (how a subject can discriminate between stimulus alternatives, i.e. object-level processes) to be differentiated from metacognitive sensitivity (a judgement on the correctness of this decision) 48 . Importantly, only comparing meta- d ′ to d ′ seems to give reliable assessments metacognitive efficiency 49 . A ratio of 1 between meta- d’ ′ and d’ ′, indicates that a subject was perfectly able to discriminate between their correct and incorrect judgements. A ratio of 0.8 suggests that 80% of the task-related sensory evidence was available for the metacognitive judgements. Table 1 provides an overview of the different types of tasks and protocols with regards to the type of metacognitive process they operationalise. These operationalisations of meta-knowledge are used in combination with brain imaging methods (functional and structural magnetic resonance imaging; fMRI; MRI) to identify brain regions associated with metacognitive activity and metacognitive abilities 10 , 50 . Alternatively, transcranial magnetic stimulation (TMS) can be used to temporarily deactivate chosen brain regions and test whether this affects metacognitive abilities in given tasks 51 , 52 .
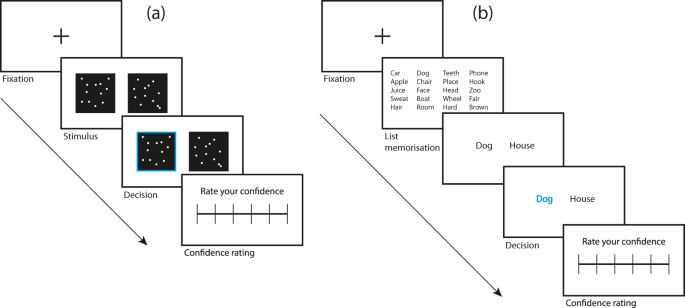
a Visual perception task: subjects choose the box containing the most (randomly generated) dots. Subjects then rate their confidence in their decision. b Memory task: subjects learn a list of words. In the next screen, they have to identify which of two words shown was present on the list. The subjects then rate their confidence in their decision.
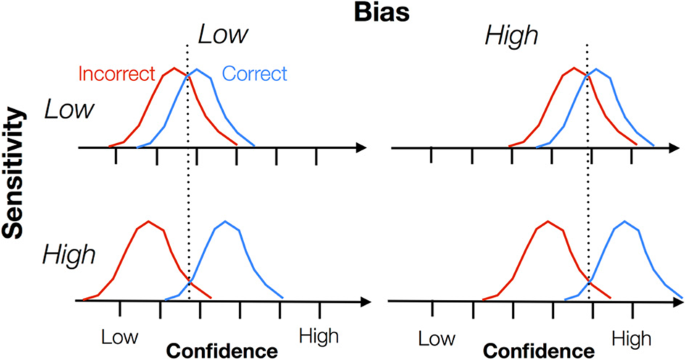
The red and blue curves represent the distribution of confidence ratings for incorrect and correct trials, respectively. A larger distance between the two curves denotes higher sensitivity. Displacement to the left and right denote biases towards low confidence (low metacognitive bias) and high confidence (high metacognitive bias), respectively (retrieved from Fig. 1 in Fleming and Lau 46 ). We repeat the disclaimer of the original authors that this figure is not a statistically accurate description of correct and incorrect responses, which are typically not normally distributed 46 , 47 .
A recent meta-analysis analysed 47 neuroimaging studies on metacognition and identified a domain-general network associated with high vs. low confidence ratings in both decision-making tasks (perception 2-AFC) and memory tasks (JOL, FOK) 11 . This network includes the medial and lateral prefrontal cortex (mPFC and lPFC, respectively), precuneus and insula. In contrast, the right anterior dorsolateral PFC (dlPFC) was specifically involved in decision-making tasks, and the bilateral parahippocampal cortex was specific to memory tasks. In addition, prospective judgements were associated with the posterior mPFC, left dlPFC and right insula, whereas retrospective judgements were associated with bilateral parahippocampal cortex and left inferior frontal gyrus. Finally, emerging evidence suggests a role of the right rostrolateral PFC (rlPFC) 53 , 54 , anterior PFC (aPFC) 44 , 45 , 55 , 56 , dorsal anterior cingulate cortex (dACC) 54 , 55 and precuneus 45 , 55 in metacognitive sensitivity (meta- d ′, ROC). In addition, several studies suggest that the aPFC relates to metacognition specifically in perception-related 2-AFC tasks, whereas the precuneus is engaged specifically in memory-related 2-AFC tasks 45 , 55 , 56 . This may suggest that metacognitive processes engage some regions in a domain-specific manner, while other regions are domain-general. For educational scientists, this could mean that some domains of metacognition may be more relevant for learning and, granted sufficient plasticity of the associated brain regions, that targeting them during interventions may show more substantial benefits. Note that rating one’s confidence and metacognitive sensitivity likely involve additional, peripheral cognitive processes instead of purely metacognitive ones. These regions are therefore associated with metacognition but not uniquely per se. Notably, a recent meta-analysis 50 suggests that domain-specific and domain-general signals may rather share common circuitry, but that their neural signature varies depending on the type of task or activity, showing that domain-generality in metacognition is complex and still needs to be better understood.
In terms of the role of metacognitive judgements on future behaviour, one study found that brain patterns associated with the desire for cognitive offloading (i.e., meta-control) partially overlap with those associated with meta-knowledge (metacognitive judgements of confidence), suggesting that meta-control is driven by either non-metacognitive, in addition to metacognitive, processes or by a combination of different domain-specific meta-knowledge processes 35 .
Executive function
In EF, processes such as error detection/monitoring and effort monitoring can be related to meta-knowledge while error correction, inhibitory control, and resource allocation can be related to meta-control 36 . To activate these processes, participants are asked to perform tasks in laboratory settings such as Flanker tasks, Stroop tasks, Demand Selection tasks and Motion Discrimination tasks (Fig. 4 ). Neural correlates of EF are investigated by having subjects perform such tasks while their brain activity is recorded with fMRI or electroencephalography (EEG). Additionally, patients with brain lesions can be tested against healthy participants to evaluate the functional role of the impaired regions 57 .
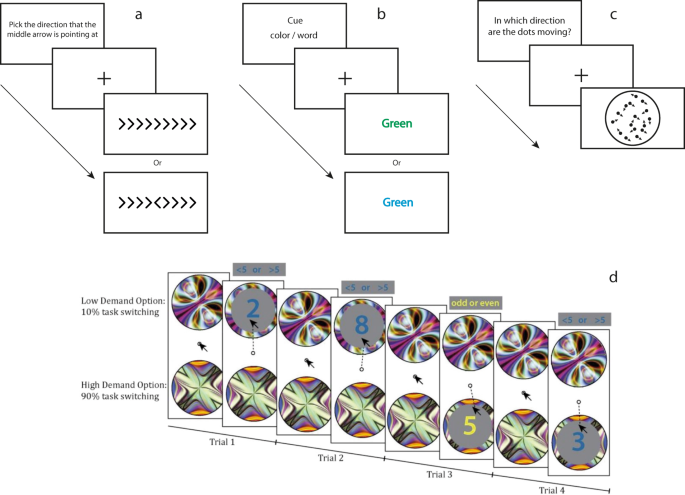
a Flanker task: subjects indicate the direction to which the arrow in the middle points. b Stroop task: subjects are presented with the name of colour printed in a colour that either matches or mismatches the name. Subjects are asked to give the name of the written colour or the printed colour. c Motion Discrimination task: subjects have to determine in which direction the dots are going with variating levels of noise. d Example of a Demand Selection task: in both options subjects have to switch between two tasks. Task one, subjects determine whether the number shown is higher or lower than 5. Task two, subjects determine whether the number is odd or even. The two options (low and high demand) differ in their degree of task switching, meaning the effort required. Subjects are allowed to switch between the two options. Note, the type of task is solely indicated by the colour of the number and that the subjects are not explicitly told about the difference in effort between the two options (retrieved from Fig. 1c in Froböse et al. 58 ).
In a review article on the neural basis of EF (in which they are defined as meta-control), Shimamura argues that a network of regions composed of the aPFC, ACC, ventrolateral PFC (vlPFC) and dlPFC is involved in the regulations of cognition 30 . These regions are not only interconnected but are also intricately connected to cortical and subcortical regions outside of the PFC. The vlPFC was shown to play an important role in “selecting and maintaining information in working memory”, whereas the dlPFC is involved in “manipulating and updating information in working memory” 30 . The ACC has been proposed to monitor cognitive conflict (e.g. in a Stroop task or a Flanker task), and the dlPFC to regulate it 58 , 59 . In particular, activity in the ACC in conflict monitoring (meta-knowledge) seems to contribute to control of cognition (meta-control) in the dlPFC 60 , 61 and to “bias behavioural decision-making toward cognitively efficient tasks and strategies” (p. 356) 62 . In a recent fMRI study, subjects performed a motion discrimination task (Fig. 4c ) 63 . After deciding on the direction of the motion, they were presented additional motion (i.e. post-decisional evidence) and then were asked to rate their confidence in their initial choice. The post-decisional evidence was encoded in the activity of the posterior medial frontal cortex (pMFC; meta-knowledge), while lateral aPFC (meta-control) modulated the impact of this evidence on subsequent confidence rating 63 . Finally, results from a meta-analysis study on cognitive control identified functional connectivity between the pMFC, associated with monitoring and informing other regions about the need for regulation, and the lPFC that would effectively regulate cognition 64 .
Online vs. offline metacognition
While the processes engaged during tasks such as those used in EF research can be considered as metacognitive in the sense that they are higher-order functions that monitor and control lower cognitive processes, scientists have argued that they are not functionally equivalent to metacognitive judgements 10 , 11 , 65 , 66 . Indeed, engaging in metacognitive judgements requires subjects to reflect on past or future activities. As such, metacognitive judgements can be considered as offline metacognitive processes. In contrast, high-order processes involved in decision-making tasks such as used in EF research are arguably largely made on the fly, or online , at a rapid pace and subjects do not need to reflect on their actions to perform them. Hence, we propose to explicitly distinguish online and offline processes. Other researchers have shared a similar view and some have proposed models for metacognition that make similar distinctions 65 , 66 , 67 , 68 . The functional difference between online and offline metacognition is supported by some evidence. For instance, event-related brain potential (ERP) studies suggest that error negativities are associated with error detection in general, whereas an increased error positivity specifically encodes error that subjects could report upon 69 , 70 . Furthermore, brain-imaging studies suggest that the MFC and ACC are involved in online meta-knowledge, while the aPFC and lPFC seem to be activated when subjects engage in more offline meta-knowledge and meta-control, respectively 63 , 71 , 72 . An overview of the different tasks can be found in Table 1 and a list of different studies on metacognition can be found in Supplementary Table 1 (organised in terms of the type of processes investigated, the protocols and brain measures used, along with the brain regions identified). Figure 5 illustrates the different brain regions associated with meta-knowledge and meta-control, distinguishing between what we consider to be online and offline processes. This distinction is often not made explicitly but it will be specifically helpful when building bridges between cognitive neuroscience and educational sciences.
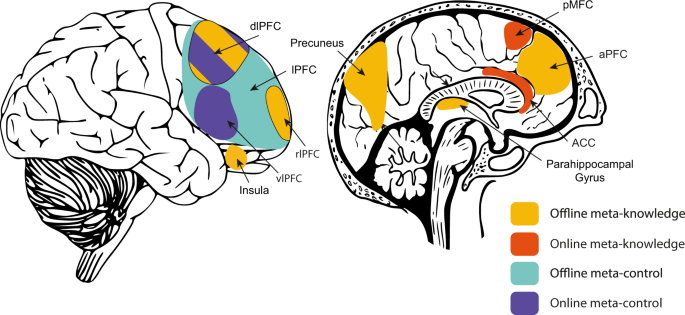
The regions are divided into online meta-knowledge and meta-control, and offline meta-knowledge and meta-control following the distinctions introduced earlier. Some regions have been reported to be related to both offline and online processes and are therefore given a striped pattern.
Training metacognition
There are extensive accounts in the literature of efforts to improve EF components such as inhibitory control, attention shifting and working memory 22 . While working memory does not directly reflect metacognitive abilities, its training is often hypothesised to improve general cognitive abilities and academic achievement. However, most meta-analyses found that training methods lead only to weak, non-lasting effects on cognitive control 73 , 74 , 75 . One meta-analysis did find evidence of near-transfer following EF training in children (in particular working memory, inhibitory control and cognitive flexibility), but found no evidence of far-transfer 20 . According to this study, training on one component leads to improved abilities in that same component but not in other EF components. Regarding adults, however, one meta-analysis suggests that EF training in general and working memory training specifically may both lead to significant near- and far-transfer effects 76 . On a neural level, a meta-analysis showed that cognitive training resulted in decreased brain activity in brain regions associated with EF 77 . According to the authors, this indicates that “training interventions reduce demands on externally focused attention” (p. 193) 77 .
With regards to meta-knowledge, several studies have reported increased task-related metacognitive abilities after training. For example, researchers found that subjects who received feedback on their metacognitive judgements regarding a perceptual decision-making task displayed better metacognitive accuracy, not only in the trained task but also in an untrained memory task 78 . Related, Baird and colleagues 79 found that a two-week mindfulness meditation training lead to enhanced meta-knowledge in the memory domain, but not the perceptual domain. The authors link these results to evidence of increased grey matter density in the aPFC in meditation practitioners.
Research on metacognition in cognitive science has mainly been studied through the lens of metacognitive judgements and EF (specifically performance monitoring and cognitive control). Meta-knowledge is commonly activated in subjects by asking them to rate their confidence in having successfully performed a task. A distinction is made between metacognitive sensitivity, metacognitive bias and metacognitive efficacy. Monitoring and regulating processes in EF are mainly operationalised with behavioural tasks such as Flanker tasks, Stroop tasks, Motion Discrimination tasks and Demand Selection tasks. In addition, metacognitive judgements can be viewed as offline processes in that they require the subject to reflect on her cognition and develop meta-representations. In contrast, EF can be considered as mostly online metacognitive processes because monitoring and regulation mostly happen rapidly without the need for reflective thinking.
Although there is some evidence for domain specificity, other studies have suggested that there is a single network of regions involved in all meta-cognitive tasks, but differentially activated in different task contexts. Comparing research on meta-knowledge and meta-control also suggest that some regions play a crucial role in both knowledge and regulation (Fig. 5 ). We have also identified a specific set of regions that are involved in either offline or online meta-knowledge. The evidence in favour of metacognitive training, while mixed, is interesting. In particular, research on offline meta-knowledge training involving self-reflection and metacognitive accuracy has shown some promising results. The regions that show structural changes after training, were those that we earlier identified as being part of the metacognition network. EF training does seem to show far-transfer effects at least in adults, but the relevance for everyday life activity is still unclear.
One major limitation of current research in metacognition is ecological validity. It is unclear to what extent the operationalisations reviewed above reflect real-life metacognition. For instance, are people who can accurately judge their performance on a behavioural task also able to accurately assess how they performed during an exam? Are people with high levels of error regulation and inhibitory control able to learn more efficiently? Note that criticism on the ecological validity of neurocognitive operationalisations extends beyond metacognition research 16 . A solution for improving validity may be to compare operationalisations of metacognition in cognitive neuroscience with the ones in educational sciences, which have shown clear links with learning in formal education. This also applies to metacognitive training.
Metacognition in educational sciences
The most popular protocols used to measure metacognition in educational sciences are self-report questionnaires or interviews, learning journals and thinking-aloud protocols 31 , 80 . During interviews, subjects are asked to answer questions regarding hypothetical situations 81 . In learning journals, students write about their learning experience and their thoughts on learning 82 , 83 . In thinking-aloud protocols, subjects are asked to verbalise their thoughts while performing a problem-solving task 80 . Each of these instruments can be used to study meta-knowledge and meta-control. For instance, one of the most widely used questionnaires, the Metacognitive Awareness Inventory (MAI) 42 , operationalises “Flavellian” metacognition and has dedicated scales for meta-knowledge and meta-control (also popular are the MSLQ 84 and LASSI 85 which operate under SRL). The meta-knowledge scale of the MAI operationalises knowledge of strategies (e.g., “ I am aware of what strategies I use when I study ”) and self-awareness (e.g., “ I am a good judge of how well I understand something ”); the meta-control scale operationalises planning (e.g., “ I set a goal before I begin a task ”) and use of learning strategies (e.g., “ I summarize what I’ve learned after I finish ”). Learning journals, self-report questionnaires and interviews involve offline metacognition. Thinking aloud, though not engaging the same degree self-reflection, also involves offline metacognition in the sense that online processes are verbalised, which necessitate offline processing (see Table 1 for an overview and Supplementary Table 2 for more details).
More recently, methodologies borrowed from cognitive neuroscience have been introduced to study EF in educational settings 22 , 86 . In particular, researchers used classic cognitive control tasks such as the Stroop task (for a meta-analysis 86 ). Most of the studied components are related to meta-control and not meta-knowledge. For instance, the BRIEF 87 is a questionnaire completed by parents and teachers which assesses different subdomains of EF: (1) inhibition, shifting, and emotional control which can be viewed as online metacognitive control, and (2) planning, organisation of materials, and monitoring, which can be viewed as offline meta-control 87 .
Assessment of metacognition is usually compared against metrics of academic performance such as grades or scores on designated tasks. A recent meta-analysis reported a weak correlation of self-report questionnaires and interviews with academic performance whereas think-aloud protocols correlated highly 88 . Offline meta-knowledge processes operationalised by learning journals were found to be positively associated with academic achievement when related to reflection on learning activities but negatively associated when related to reflection on learning materials, indicating that the type of reflection is important 89 . EF have been associated with abilities in mathematics (mainly) and reading comprehension 86 . However, the literature points towards contrary directions as to what specific EF component is involved in academic achievement. This may be due to the different groups that were studied, to different operationalisations or to different theoretical underpinnings for EF 86 . For instance, online and offline metacognitive processes, which are not systematically distinguished in the literature, may play different roles in academic achievement. Moreover, the bulk of research focussed on young children with few studies on adolescents 86 and EF may play a role at varying extents at different stages of life.
A critical question in educational sciences is that of the nature of the relationship between metacognition and academic achievement to understand whether learning at school can be enhanced by training metacognitive abilities. Does higher metacognition lead to higher academic achievement? Do these features evolve in parallel? Developmental research provides valuable insights into the formation of metacognitive abilities that can inform training designs in terms of what aspect of metacognition should be supported and the age at which interventions may yield the best results. First, meta-knowledge seems to emerge around the age of 5, meta-control around 8, and both develop over the years 90 , with evidence for the development of meta-knowledge into adolescence 91 . Furthermore, current theories propose that meta-knowledge abilities are initially highly domain-dependent and gradually become more domain-independent as knowledge and experience are acquired and linked between domains 32 . Meta-control is believed to evolve in a similar fashion 90 , 92 .
Common methods used to train offline metacognition are direct instruction of metacognition, metacognitive prompts and learning journals. In addition, research has been done on the use of (self-directed) feedback as a means to induce self-reflection in students, mainly in computer-supported settings 93 . Interestingly, learning journals appear to be used for both assessing and fostering metacognition. Metacognitive instruction consists of teaching learners’ strategies to “activate” their metacognition. Metacognitive prompts most often consist of text pieces that are sent at specific times and that trigger reflection (offline meta-knowledge) on learning behaviour in the form of a question, hint or reminder.
Meta-analyses have investigated the effects of direct metacognitive instruction on students’ use of learning strategies and academic outcomes 18 , 94 , 95 . Their findings show that metacognitive instruction can have a positive effect on learning abilities and achievement within a population ranging from primary schoolers to university students. In particular, interventions lead to the highest effect sizes when they both (i) instructed a combination of metacognitive strategies with an emphasis on planning strategies (offline meta-control) and (ii) “provided students with knowledge about strategies” (offline meta-knowledge) and “illustrated the benefits of applying the trained strategies, or even stimulated metacognitive reasoning” (p.114) 18 . The longer the duration of the intervention, the more effective they were. The strongest effects on academic performance were observed in the context of mathematics, followed by reading and writing.
While metacognitive prompts and learning journals make up the larger part of the literature on metacognitive training 96 , meta-analyses that specifically investigate their effectiveness have yet to be performed. Nonetheless, evidence suggests that such interventions can be successful. Researchers found that metacognitive prompts fostered the use of metacognitive strategies (offline meta-control) and that the combination of cognitive and metacognitive prompts improved learning outcomes 97 . Another experiment showed that students who received metacognitive prompts performed more metacognitive activities inside the learning environment and displayed better transfer performance immediately after the intervention 98 . A similar study using self-directed prompts showed enhanced transfer performance that was still observable 3 weeks after the intervention 99 .
Several studies suggest that learning journals can positively enhance metacognition. Subjects who kept a learning journal displayed stronger high meta-control and meta-knowledge on learning tasks and tended to reach higher academic outcomes 100 , 101 , 102 . However, how the learning journal is used seems to be critical; good instructions are crucial 97 , 103 , and subjects who simply summarise their learning activity benefit less from the intervention than subjects who reflect about their knowledge, learning and learning goals 104 . An overview of studies using learning journals and metacognitive prompts to train metacognition can be found in Supplementary Table 3 .
In recent years, educational neuroscience researchers have tried to determine whether training and improvements in EF can lead to learning facilitation and higher academic achievement. Training may consist of having students continually perform behavioural tasks either in the lab, at home, or at school. Current evidence in favour of training EF is mixed, with only anecdotal evidence for positive effects 105 . A meta-analysis did not show evidence for a causal relationship between EF and academic achievement 19 , but suggested that the relationship is bidirectional, meaning that the two are “mutually supportive” 106 .
A recent review article has identified several gaps and shortcoming in the literature on metacognitive training 96 . Overall, research in metacognitive training has been mainly invested in developing learners’ meta-control rather than meta-knowledge. Furthermore, most of the interventions were done in the context of science learning. Critically, there appears to be a lack of studies that employed randomised control designs, such that the effects of metacognitive training intervention are often difficult to evaluate. In addition, research overwhelmingly investigated metacognitive prompts and learning journals in adults 96 , while interventions on EF mainly focused on young children 22 . Lastly, meta-analyses evaluating the effectiveness of metacognitive training have so far focused on metacognitive instruction on children. There is thus a clear disbalance between the meta-analyses performed and the scope of the literature available.
An important caveat of educational sciences research is that metacognition is not typically framed in terms of online and offline metacognition. Therefore, it can be unclear whether protocols operationalise online or offline processes and whether interventions tend to benefit more online or offline metacognition. There is also confusion in terms of what processes qualify as EF and definitions of it vary substantially 86 . For instance, Clements and colleagues mention work on SRL to illustrate research in EF in relation to academic achievement but the two spawn from different lines of research, one rooted in metacognition and socio-cognitive theory 31 and the other in the cognitive (neuro)science of decision-making. In addition, the MSLQ, as discussed above, assesses offline metacognition along with other components relevant to SRL, whereas EF can be mainly understood as online metacognition (see Table 1 ), which on the neural level may rely on different circuitry.
Investigating offline metacognition tends to be carried out in school settings whereas evaluating EF (e.g., Stroop task, and BRIEF) is performed in the lab. Common to all protocols for offline metacognition is that they consist of a form of self-report from the learner, either during the learning activity (thinking-aloud protocols) or after the learning activity (questionnaires, interviews and learning journals). Questionnaires are popular protocols due to how easy they are to administer but have been criticised to provide biased evaluations of metacognitive abilities. In contrast, learning journals evaluate the degree to which learners engage in reflective thinking and may therefore be less prone to bias. Lastly, it is unclear to what extent thinking-aloud protocols are sensitive to online metacognitive processes, such as on-the-fly error correction and effort regulation. The strength of the relationship between metacognitive abilities and academic achievement varies depending on how metacognition is operationalised. Self-report questionnaires and interviews are weakly related to achievement whereas thinking-aloud protocols and EF are strongly related to it.
Based on the well-documented relationship between metacognition and academic achievement, educational scientists hypothesised that fostering metacognition may improve learning and academic achievement, and thus performed metacognitive training interventions. The most prevalent training protocols are direct metacognitive instruction, learning journals, and metacognitive prompts, which aim to induce and foster offline metacognitive processes such as self-reflection, planning and selecting learning strategies. In addition, researchers have investigated whether training EF, either through tasks or embedded in the curriculum, results in higher academic proficiency and achievement. While a large body of evidence suggests that metacognitive instruction, learning journals and metacognitive prompts can successfully improve academic achievement, interventions designed around EF training show mixed results. Future research investigating EF training in different age categories may clarify this situation. These various degrees of success of interventions may indicate that offline metacognition is more easily trainable than online metacognition and plays a more important role in educational settings. Investigating the effects of different methods, offline and online, on the neural level, may provide researchers with insights into the trainability of different metacognitive processes.
In this article, we reviewed the literature on metacognition in educational sciences and cognitive neuroscience with the aim to investigate gaps in current research and propose ways to address them through the exchange of insights between the two disciplines and interdisciplinary approaches. The main aspects analysed were operational definitions of metacognition and metacognitive training, through the lens of metacognitive knowledge and metacognitive control. Our review also highlighted an additional construct in the form of the distinction between online metacognition (on the fly and largely automatic) and offline metacognition (slower, reflective and requiring meta-representations). In cognitive neuroscience, research has focused on metacognitive judgements (mainly offline) and EF (mainly online). Metacognition is operationalised with tasks carried out in the lab and are mapped onto brain functions. In contrast, research in educational sciences typically measures metacognition in the context of learning activities, mostly in schools and universities. More recently, EF has been studied in educational settings to investigate its role in academic achievement and whether training it may benefit learning. Evidence on the latter is however mixed. Regarding metacognitive training in general, evidence from both disciplines suggests that interventions fostering learners’ self-reflection and knowledge of their learning behaviour (i.e., offline meta-knowledge) may best benefit them and increase academic achievement.
We focused on four aspects of research that could benefit from an interdisciplinary approach between the two areas: (i) validity and reliability of research protocols, (ii) under-researched dimensions of metacognition, (iii) metacognitive training, and (iv) domain-specificity vs. domain generality of metacognitive abilities. To tackle these issue, we propose four avenues for integrated research: (i) investigate the degree to which different protocols relate to similar or different metacognitive constructs, (ii) implement designs and perform experiments to identify neural substrates necessary for offline meta-control by for example borrowing protocols used in educational sciences, (iii) study the effects of (offline) meta-knowledge training on the brain, and (iv) perform developmental research in the metacognitive brain and compare it with the existing developmental literature in educational sciences regarding the domain-generality of metacognitive processes and metacognitive abilities.
First, neurocognitive research on metacognitive judgements has developed robust operationalisations of offline meta-knowledge. However, these operationalisations often consist of specific tasks (e.g., 2-AFC) carried out in the lab. These tasks are often very narrow and do not resemble the challenges and complexities of behaviours associated with learning in schools and universities. Thus, one may question to what extent they reflect real-life metacognition, and to what extent protocols developed in educational sciences and cognitive neuroscience actually operationalise the same components of metacognition. We propose that comparing different protocols from both disciplines that are, a priori, operationalising the same types of metacognitive processes can help evaluate the ecological validity of protocols used in cognitive neuroscience, and allow for more holistic assessments of metacognition, provided that it is clear which protocol assesses which construct. Degrees of correlation between different protocols, within and between disciplines, may allow researchers to assess to what extent they reflect the same metacognitive constructs and also identify what protocols are most appropriate to study a specific construct. For example, a relation between meta- d ′ metacognitive sensitivity in a 2-AFC task and the meta-knowledge subscale of the MAI, would provide external validity to the former. Moreover, educational scientists would be provided with bias-free tools to assess metacognition. These tools may enable researchers to further investigate to what extent metacognitive bias, sensitivity and efficiency each play a role in education settings. In contrast, a low correlation may highlight a difference in domain between the two measures of metacognition. For instance, metacognitive judgements in brain research are made in isolated behaviour, and meta-d’ can thus be viewed to reflect “local” metacognitive sensitivity. It is also unclear to what extent processes involved in these decision-making tasks cover those taking place in a learning environment. When answering self-reported questionnaires, however, subjects make metacognitive judgements on a large set of (learning) activities, and the measures may thus resemble more “global” or domain-general metacognitive sensitivity. In addition, learners in educational settings tend to receive feedback — immediate or delayed — on their learning activities and performance, which is generally not the case for cognitive neuroscience protocols. Therefore, investigating metacognitive judgements in the presence of performance or social feedback may allow researchers to better understand the metacognitive processes at play in educational settings. Devising a global measure of metacognition in the lab by aggregating subjects’ metacognitive abilities in different domains or investigating to what extent local metacognition may affect global metacognition could improve ecological validity significantly. By investigating the neural correlates of educational measures of metacognition, researchers may be able to better understand to what extent the constructs studied in the two disciplines are related. It is indeed possible that, though weakly correlated, the meta-knowledge scale of the MAI and meta-d’ share a common neural basis.
Second, our review highlights gaps in the literature of both disciplines regarding the research of certain types of metacognitive processes. There is a lack of research in offline meta-control (or strategic regulation of cognition) in neuroscience, whereas this construct is widely studied in educational sciences. More specifically, while there exists research on EF related to planning (e.g. 107 ), common experimental designs make it hard to disentangle online from offline metacognitive processes. A few studies have implemented subject reports (e.g., awareness of error or desire for reminders) to pin-point the neural substrates specifically involved in offline meta-control and the current evidence points at a role of the lPFC. More research implementing similar designs may clarify this construct. Alternatively, researchers may exploit educational sciences protocols, such as self-report questionnaires, learning journals, metacognitive prompts and feedback to investigate offline meta-control processes in the brain and their relation to academic proficiency and achievement.
Third, there is only one study known to us on the training of meta-knowledge in the lab 78 . In contrast, meta-knowledge training in educational sciences have been widely studied, in particular with metacognitive prompts and learning journals, although a systematic review would be needed to identify the benefits for learning. Relative to cognitive neuroscience, studies suggest that offline meta-knowledge trained in and outside the lab (i.e., metacognitive judgements and meditation, respectively) transfer to meta-knowledge in other lab tasks. The case of meditation is particularly interesting since meditation has been demonstrated to beneficiate varied aspects of everyday life 108 . Given its importance for efficient regulation of cognition, training (offline) meta-knowledge may present the largest benefits to academic achievement. Hence, it is important to investigate development in the brain relative to meta-knowledge training. Evidence on metacognitive training in educational sciences tends to suggest that offline metacognition is more “plastic” and may therefore benefit learning more than online metacognition. Furthermore, it is important to have a good understanding of the developmental trajectory of metacognitive abilities — not only on a behavioural level but also on a neural level — to identify critical periods for successful training. Doing so would also allow researchers to investigate the potential differences in terms of plasticity that we mention above. Currently, the developmental trajectory of metacognition is under-studied in cognitive neuroscience with only one study that found an overlap between the neural correlates of metacognition in adults and children 109 . On a side note, future research could explore the potential role of genetic factors in metacognitive abilities to better understand to what extent and under what constraints they can be trained.
Fourth, domain-specific and domain-general aspects of metacognitive processes should be further investigated. Educational scientists have studied the development of metacognition in learners and have concluded that metacognitive abilities are domain-specific at the beginning (meaning that their quality depends on the type of learning activity, like mathematics vs. writing) and progressively evolve towards domain-general abilities as knowledge and expertise increase. Similarly, neurocognitive evidence points towards a common network for (offline) metacognitive knowledge which engages the different regions at varying degrees depending on the domain of the activity (i.e., perception, memory, etc.). Investigating this network from a developmental perspective and comparing findings with the existing behavioural literature may improve our understanding of the metacognitive brain and link the two bodies of evidence. It may also enable researchers to identify stages of life more suitable for certain types of metacognitive intervention.
Dunlosky, J. & Metcalfe, J. Metacognition (SAGE Publications, 2008).
Pintrich, P. R. The role of metacognitive knowledge in learning, teaching, and assessing. Theory Into Pract. 41 , 219–225 (2002).
Article Google Scholar
Zimmerman, B. J. Self-regulated learning and academic achievement: an overview. Educ. Psychol. 25 , 3–17 (1990).
Zimmerman, B. J. & Schunk, D. H. Self-Regulated Learning and Academic Achievement: Theoretical Perspectives (Routledge, 2001).
Baker, L. & Brown, A. L. Metacognitive Skills and Reading. In Handbook of Reading Research Vol. 1 (ed. Pearson, P. D.) 353–395 (Longman, 1984).
Mckeown, M. G. & Beck, I. L. The role of metacognition in understanding and supporting reading comprehension. In Handbook of Metacognition in Education (eds Hacker, D. J., Dunlosky, J. & Graesser, A. C.) 19–37 (Routledge, 2009).
Desoete, A., Roeyers, H. & Buysse, A. Metacognition and mathematical problem solving in grade 3. J. Learn. Disabil. 34 , 435–447 (2001).
Article CAS PubMed Google Scholar
Veenman, M., Kok, R. & Blöte, A. W. The relation between intellectual and metacognitive skills in early adolescence. Instructional Sci. 33 , 193–211 (2005).
Harris, K. R., Graham, S., Brindle, M. & Sandmel, K. Metacognition and children’s writing. In Handbook of metacognition in education 131–153 (Routledge, 2009).
Fleming, S. M. & Dolan, R. J. The neural basis of metacognitive ability. Philos. Trans. R. Soc. B 367 , 1338–1349 (2012).
Vaccaro, A. G. & Fleming, S. M. Thinking about thinking: a coordinate-based meta-analysis of neuroimaging studies of metacognitive judgements. Brain Neurosci. Adv. 2 , 10.1177%2F2398212818810591 (2018).
Ferrari, M. What can neuroscience bring to education? Educ. Philos. Theory 43 , 31–36 (2011).
Zadina, J. N. The emerging role of educational neuroscience in education reform. Psicol. Educ. 21 , 71–77 (2015).
Meulen, A., van der, Krabbendam, L. & Ruyter, Dde Educational neuroscience: its position, aims and expectations. Br. J. Educ. Stud. 63 , 229–243 (2015).
Varma, S., McCandliss, B. D. & Schwartz, D. L. Scientific and pragmatic challenges for bridging education and neuroscience. Educ. Res. 37 , 140–152 (2008).
van Atteveldt, N., van Kesteren, M. T. R., Braams, B. & Krabbendam, L. Neuroimaging of learning and development: improving ecological validity. Frontline Learn. Res. 6 , 186–203 (2018).
Article PubMed PubMed Central Google Scholar
Hruby, G. G. Three requirements for justifying an educational neuroscience. Br. J. Educ. Psychol. 82 , 1–23 (2012).
Article PubMed Google Scholar
Dignath, C., Buettner, G. & Langfeldt, H.-P. How can primary school students learn self-regulated learning strategies most effectively?: A meta-analysis on self-regulation training programmes. Educ. Res. Rev. 3 , 101–129 (2008).
Jacob, R. & Parkinson, J. The potential for school-based interventions that target executive function to improve academic achievement: a review. Rev. Educ. Res. 85 , 512–552 (2015).
Kassai, R., Futo, J., Demetrovics, Z. & Takacs, Z. K. A meta-analysis of the experimental evidence on the near- and far-transfer effects among children’s executive function skills. Psychol. Bull. 145 , 165–188 (2019).
Roebers, C. M. Executive function and metacognition: towards a unifying framework of cognitive self-regulation. Dev. Rev. 45 , 31–51 (2017).
Clements, D. H., Sarama, J. & Germeroth, C. Learning executive function and early mathematics: directions of causal relations. Early Child. Res. Q. 36 , 79–90 (2016).
Nelson, T. O. & Narens, L. Metamemory. In Perspectives on the development of memory and cognition (ed. R. V. Kail & J. W. Hag) 3–33 (Hillsdale, N.J.: Erlbaum, 1977).
Baird, J. R. Improving learning through enhanced metacognition: a classroom study. Eur. J. Sci. Educ. 8 , 263–282 (1986).
Flavell, J. H. & Wellman, H. M. Metamemory (1975).
Flavell, J. H. Metacognition and cognitive monitoring: a new area of cognitive–developmental inquiry. Am. Psychol. 34 , 906 (1979).
Livingston, J. A. Metacognition: An Overview. (2003).
Nelson, T. O. Metamemory: a theoretical framework and new findings. In Psychology of Learning and Motivation Vol. 26 (ed. Bower, G. H.) 125–173 (Academic Press, 1990).
Nelson, T. O. & Narens, L. Why investigate metacognition. In Metacognition: Knowing About Knowing (eds Metcalfe, J. & Shimamura, A. P.) 1–25 (MIT Press, 1994).
Shimamura, A. P. A Neurocognitive approach to metacognitive monitoring and control. In Handbook of Metamemory and Memory (eds Dunlosky, J. & Bjork, R. A.) (Routledge, 2014).
Dinsmore, D. L., Alexander, P. A. & Loughlin, S. M. Focusing the conceptual lens on metacognition, self-regulation, and self-regulated learning. Educ. Psychol. Rev. 20 , 391–409 (2008).
Borkowski, J. G., Chan, L. K. & Muthukrishna, N. A process-oriented model of metacognition: links between motivation and executive functioning. In (Gregory Schraw & James C. Impara) Issues in the Measurement of Metacognition 1–42 (Buros Institute of Mental Measurements, 2000).
Risko, E. F. & Gilbert, S. J. Cognitive offloading. Trends Cogn. Sci. 20 , 676–688 (2016).
Gilbert, S. J. et al. Optimal use of reminders: metacognition, effort, and cognitive offloading. J. Exp. Psychol. 149 , 501 (2020).
Boldt, A. & Gilbert, S. Distinct and overlapping neural correlates of metacognitive monitoring and metacognitive control. Preprint at bioRxiv https://psyarxiv.com/3dz9b/ (2020).
Fernandez-Duque, D., Baird, J. A. & Posner, M. I. Executive attention and metacognitive regulation. Conscious Cogn. 9 , 288–307 (2000).
Baker, L., Zeliger-Kandasamy, A. & DeWyngaert, L. U. Neuroimaging evidence of comprehension monitoring. Psihol. teme 23 , 167–187 (2014).
Google Scholar
Schwartz, B. L. Sources of information in metamemory: Judgments of learning and feelings of knowing. Psychon. Bull. Rev. 1 , 357–375 (1994).
Nelson, T. O. Metamemory, psychology of. In International Encyclopedia of the Social & Behavioral Sciences (eds Smelser, N. J. & Baltes, P. B.) 9733–9738 (Pergamon, 2001).
Hart, J. T. Memory and the feeling-of-knowing experience. J. Educ. Psychol. 56 , 208 (1965).
Arbuckle, T. Y. & Cuddy, L. L. Discrimination of item strength at time of presentation. J. Exp. Psychol. 81 , 126 (1969).
Fechner, G. T. Elemente der Psychophysik (Breitkopf & Härtel, 1860).
Rouault, M., Seow, T., Gillan, C. M. & Fleming, S. M. Psychiatric symptom dimensions are associated with dissociable shifts in metacognition but not task performance. Biol. Psychiatry 84 , 443–451 (2018).
Fleming, S. M., Weil, R. S., Nagy, Z., Dolan, R. J. & Rees, G. Relating introspective accuracy to individual differences in brain structure. Science 329 , 1541–1543 (2010).
Article CAS PubMed PubMed Central Google Scholar
McCurdy, L. Y. et al. Anatomical coupling between distinct metacognitive systems for memory and visual perception. J. Neurosci. 33 , 1897–1906 (2013).
Fleming, S. M. & Lau, H. C. How to measure metacognition. Front. Hum. Neurosci. 8 https://doi.org/10.3389/fnhum.2014.00443 (2014).
Galvin, S. J., Podd, J. V., Drga, V. & Whitmore, J. Type 2 tasks in the theory of signal detectability: discrimination between correct and incorrect decisions. Psychon. Bull. Rev. 10 , 843–876 (2003).
Metcalfe, J. & Schwartz, B. L. The ghost in the machine: self-reflective consciousness and the neuroscience of metacognition. In (eds Dunlosky, J. & Tauber, S. K.) Oxford Handbook of Metamemory 407–424 (Oxford University Press, 2016).
Maniscalco, B. & Lau, H. A signal detection theoretic approach for estimating metacognitive sensitivity from confidence ratings. Conscious Cognition 21 , 422–430 (2012).
Rouault, M., McWilliams, A., Allen, M. G. & Fleming, S. M. Human metacognition across domains: insights from individual differences and neuroimaging. Personal. Neurosci. 1 https://doi.org/10.1017/pen.2018.16 (2018).
Rounis, E., Maniscalco, B., Rothwell, J. C., Passingham, R. E. & Lau, H. Theta-burst transcranial magnetic stimulation to the prefrontal cortex impairs metacognitive visual awareness. Cogn. Neurosci. 1 , 165–175 (2010).
Ye, Q., Zou, F., Lau, H., Hu, Y. & Kwok, S. C. Causal evidence for mnemonic metacognition in human precuneus. J. Neurosci. 38 , 6379–6387 (2018).
Fleming, S. M., Huijgen, J. & Dolan, R. J. Prefrontal contributions to metacognition in perceptual decision making. J. Neurosci. 32 , 6117–6125 (2012).
Morales, J., Lau, H. & Fleming, S. M. Domain-general and domain-specific patterns of activity supporting metacognition in human prefrontal cortex. J. Neurosci. 38 , 3534–3546 (2018).
Baird, B., Smallwood, J., Gorgolewski, K. J. & Margulies, D. S. Medial and lateral networks in anterior prefrontal cortex support metacognitive ability for memory and perception. J. Neurosci. 33 , 16657–16665 (2013).
Fleming, S. M., Ryu, J., Golfinos, J. G. & Blackmon, K. E. Domain-specific impairment in metacognitive accuracy following anterior prefrontal lesions. Brain 137 , 2811–2822 (2014).
Baldo, J. V., Shimamura, A. P., Delis, D. C., Kramer, J. & Kaplan, E. Verbal and design fluency in patients with frontal lobe lesions. J. Int. Neuropsychol. Soc. 7 , 586–596 (2001).
Froböse, M. I. et al. Catecholaminergic modulation of the avoidance of cognitive control. J. Exp. Psychol. Gen. 147 , 1763 (2018).
Botvinick, M. M., Braver, T. S., Barch, D. M., Carter, C. S. & Cohen, J. D. Conflict monitoring and cognitive control. Psychol. Rev. 108 , 624 (2001).
Kerns, J. G. et al. Anterior cingulate conflict monitoring and adjustments in control. Science 303 , 1023–1026 (2004).
Yeung, N. Conflict monitoring and cognitive control. In The Oxford Handbook of Cognitive Neuroscience: The Cutting Edges Vol. 2 (eds Ochsner, K. N. & Kosslyn, S.) 275–299 (Oxford University Press, 2014).
Botvinick, M. M. Conflict monitoring and decision making: reconciling two perspectives on anterior cingulate function. Cogn. Affect. Behav. Neurosci. 7 , 356–366 (2007).
Fleming, S. M., van der Putten, E. J. & Daw, N. D. Neural mediators of changes of mind about perceptual decisions. Nat. Neurosci. 21 , 617–624 (2018).
Ridderinkhof, K. R., Ullsperger, M., Crone, E. A. & Nieuwenhuis, S. The role of the medial frontal cortex in cognitive control. Science 306 , 443–447 (2004).
Koriat, A. The feeling of knowing: some metatheoretical implications for consciousness and control. Conscious Cogn. 9 , 149–171 (2000).
Thompson, V. A., Evans, J. & Frankish, K. Dual process theories: a metacognitive perspective. Ariel 137 , 51–43 (2009).
Arango-Muñoz, S. Two levels of metacognition. Philosophia 39 , 71–82 (2011).
Shea, N. et al. Supra-personal cognitive control and metacognition. Trends Cogn. Sci. 18 , 186–193 (2014).
Nieuwenhuis, S., Ridderinkhof, K. R., Blom, J., Band, G. P. & Kok, A. Error-related brain potentials are differentially related to awareness of response errors: evidence from an antisaccade task. Psychophysiology 38 , 752–760 (2001).
Overbeek, T. J., Nieuwenhuis, S. & Ridderinkhof, K. R. Dissociable components of error processing: on the functional significance of the Pe vis-à-vis the ERN/Ne. J. Psychophysiol. 19 , 319–329 (2005).
McGuire, J. T. & Botvinick, M. M. Prefrontal cortex, cognitive control, and the registration of decision costs. Proc. Natl Acad. Sci. USA 107 , 7922–7926 (2010).
Hester, R., Foxe, J. J., Molholm, S., Shpaner, M. & Garavan, H. Neural mechanisms involved in error processing: a comparison of errors made with and without awareness. Neuroimage 27 , 602–608 (2005).
Melby-Lervåg, M. & Hulme, C. Is working memory training effective? A meta-analytic review. Dev. Psychol. 49 , 270 (2013).
Soveri, A., Antfolk, J., Karlsson, L., Salo, B. & Laine, M. Working memory training revisited: a multi-level meta-analysis of n-back training studies. Psychon. Bull. Rev. 24 , 1077–1096 (2017).
Schwaighofer, M., Fischer, F. & Bühner, M. Does working memory training transfer? A meta-analysis including training conditions as moderators. Educ. Psychol. 50 , 138–166 (2015).
Karbach, J. & Verhaeghen, P. Making working memory work: a meta-analysis of executive-control and working memory training in older adults. Psychol. Sci. 25 , 2027–2037 (2014).
Patel, R., Spreng, R. N. & Turner, G. R. Functional brain changes following cognitive and motor skills training: a quantitative meta-analysis. Neurorehabil Neural Repair 27 , 187–199 (2013).
Carpenter, J. et al. Domain-general enhancements of metacognitive ability through adaptive training. J. Exp. Psychol. 148 , 51–64 (2019).
Baird, B., Mrazek, M. D., Phillips, D. T. & Schooler, J. W. Domain-specific enhancement of metacognitive ability following meditation training. J. Exp. Psychol. 143 , 1972 (2014).
Winne, P. H. & Perry, N. E. Measuring self-regulated learning. In Handbook of Self-Regulation (eds Boekaerts, M., Pintrich, P. R. & Zeidner, M.) Ch. 16, 531–566 (Academic Press, 2000).
Zimmerman, B. J. & Martinez-Pons, M. Development of a structured interview for assessing student use of self-regulated learning strategies. Am. Educ. Res. J. 23 , 614–628 (1986).
Park, C. Engaging students in the learning process: the learning journal. J. Geogr. High. Educ. 27 , 183–199 (2003).
Article CAS Google Scholar
Harrison, G. M. & Vallin, L. M. Evaluating the metacognitive awareness inventory using empirical factor-structure evidence. Metacogn. Learn. 13 , 15–38 (2018).
Pintrich, P. R., Smith, D. A. F., Garcia, T. & Mckeachie, W. J. Reliability and predictive validity of the motivated strategies for learning questionnaire (MSLQ). Educ. Psychol. Meas. 53 , 801–813 (1993).
Prevatt, F., Petscher, Y., Proctor, B. E., Hurst, A. & Adams, K. The revised Learning and Study Strategies Inventory: an evaluation of competing models. Educ. Psychol. Meas. 66 , 448–458 (2006).
Baggetta, P. & Alexander, P. A. Conceptualization and operationalization of executive function. Mind Brain Educ. 10 , 10–33 (2016).
Gioia, G. A., Isquith, P. K., Guy, S. C. & Kenworthy, L. Test review behavior rating inventory of executive function. Child Neuropsychol. 6 , 235–238 (2000).
Ohtani, K. & Hisasaka, T. Beyond intelligence: a meta-analytic review of the relationship among metacognition, intelligence, and academic performance. Metacogn. Learn. 13 , 179–212 (2018).
Dianovsky, M. T. & Wink, D. J. Student learning through journal writing in a general education chemistry course for pre-elementary education majors. Sci. Educ. 96 , 543–565 (2012).
Veenman, M. V. J., Van Hout-Wolters, B. H. A. M. & Afflerbach, P. Metacognition and learning: conceptual and methodological considerations. Metacogn Learn. 1 , 3–14 (2006).
Weil, L. G. et al. The development of metacognitive ability in adolescence. Conscious Cogn. 22 , 264–271 (2013).
Veenman, M. & Spaans, M. A. Relation between intellectual and metacognitive skills: Age and task differences. Learn. Individ. Differ. 15 , 159–176 (2005).
Verbert, K. et al. Learning dashboards: an overview and future research opportunities. Personal. Ubiquitous Comput. 18 , 1499–1514 (2014).
Dignath, C. & Büttner, G. Components of fostering self-regulated learning among students. A meta-analysis on intervention studies at primary and secondary school level. Metacogn. Learn. 3 , 231–264 (2008).
Hattie, J., Biggs, J. & Purdie, N. Effects of learning skills interventions on student learning: a meta-analysis. Rev. Educ. Res. 66 , 99–136 (1996).
Zohar, A. & Barzilai, S. A review of research on metacognition in science education: current and future directions. Stud. Sci. Educ. 49 , 121–169 (2013).
Berthold, K., Nückles, M. & Renkl, A. Do learning protocols support learning strategies and outcomes? The role of cognitive and metacognitive prompts. Learn. Instr. 17 , 564–577 (2007).
Bannert, M. & Mengelkamp, C. Scaffolding hypermedia learning through metacognitive prompts. In International Handbook of Metacognition and Learning Technologies Vol. 28 (eds Azevedo, R. & Aleven, V.) 171–186 (Springer New York, 2013).
Bannert, M., Sonnenberg, C., Mengelkamp, C. & Pieger, E. Short- and long-term effects of students’ self-directed metacognitive prompts on navigation behavior and learning performance. Comput. Hum. Behav. 52 , 293–306 (2015).
McCrindle, A. R. & Christensen, C. A. The impact of learning journals on metacognitive and cognitive processes and learning performance. Learn. Instr. 5 , 167–185 (1995).
Connor-Greene, P. A. Making connections: evaluating the effectiveness of journal writing in enhancing student learning. Teach. Psychol. 27 , 44–46 (2000).
Wong, B. Y. L., Kuperis, S., Jamieson, D., Keller, L. & Cull-Hewitt, R. Effects of guided journal writing on students’ story understanding. J. Educ. Res. 95 , 179–191 (2002).
Nückles, M., Schwonke, R., Berthold, K. & Renkl, A. The use of public learning diaries in blended learning. J. Educ. Media 29 , 49–66 (2004).
Cantrell, R. J., Fusaro, J. A. & Dougherty, E. A. Exploring the effectiveness of journal writing on learning social studies: a comparative study. Read. Psychol. 21 , 1–11 (2000).
Blair, C. Executive function and early childhood education. Curr. Opin. Behav. Sci. 10 , 102–107 (2016).
Clements, D. H., Sarama, J., Unlu, F. & Layzer, C. The Efficacy of an Intervention Synthesizing Scaffolding Designed to Promote Self-Regulation with an Early Mathematics Curriculum: Effects on Executive Function (Society for Research on Educational Effectiveness, 2012).
Newman, S. D., Carpenter, P. A., Varma, S. & Just, M. A. Frontal and parietal participation in problem solving in the Tower of London: fMRI and computational modeling of planning and high-level perception. Neuropsychologia 41 , 1668–1682 (2003).
Sedlmeier, P. et al. The psychological effects of meditation: a meta-analysis. Psychol. Bull. 138 , 1139 (2012).
Bellon, E., Fias, W., Ansari, D. & Smedt, B. D. The neural basis of metacognitive monitoring during arithmetic in the developing brain. Hum. Brain Mapp. 41 , 4562–4573 (2020).
Download references
Acknowledgements
We would like to thank the University of Amsterdam for supporting this research through the Interdisciplinary Doctorate Agreement grant. W.v.d.B. is further supported by the Jacobs Foundation, European Research Council (grant no. ERC-2018-StG-803338), the European Union Horizon 2020 research and innovation programme (grant no. DiGYMATEX-870578), and the Netherlands Organization for Scientific Research (grant no. NWO-VIDI 016.Vidi.185.068).
Author information
Authors and affiliations.
Informatics Institute, University of Amsterdam, Amsterdam, the Netherlands
Damien S. Fleur & Bert Bredeweg
Departement of Psychology, University of Amsterdam, Amsterdam, the Netherlands
Damien S. Fleur & Wouter van den Bos
Faculty of Education, Amsterdam University of Applied Sciences, Amsterdam, the Netherlands
Bert Bredeweg
Center for Adaptive Rationality, Max Planck Institute for Human Development, Berlin, Germany
Wouter van den Bos
You can also search for this author in PubMed Google Scholar
Contributions
D.S.F., B.B. and W.v.d.B. conceived the main conceptual idea of this review article. D.S.F. wrote the manuscript with inputs from and under the supervision of B.B. and W.v.d.B.
Corresponding author
Correspondence to Damien S. Fleur .
Ethics declarations
Competing interests.
The authors declare no competing interests.
Additional information
Publisher’s note Springer Nature remains neutral with regard to jurisdictional claims in published maps and institutional affiliations.
Supplementary information
Supplementary materials, rights and permissions.
Open Access This article is licensed under a Creative Commons Attribution 4.0 International License, which permits use, sharing, adaptation, distribution and reproduction in any medium or format, as long as you give appropriate credit to the original author(s) and the source, provide a link to the Creative Commons license, and indicate if changes were made. The images or other third party material in this article are included in the article’s Creative Commons license, unless indicated otherwise in a credit line to the material. If material is not included in the article’s Creative Commons license and your intended use is not permitted by statutory regulation or exceeds the permitted use, you will need to obtain permission directly from the copyright holder. To view a copy of this license, visit http://creativecommons.org/licenses/by/4.0/ .
Reprints and permissions
About this article
Cite this article.
Fleur, D.S., Bredeweg, B. & van den Bos, W. Metacognition: ideas and insights from neuro- and educational sciences. npj Sci. Learn. 6 , 13 (2021). https://doi.org/10.1038/s41539-021-00089-5
Download citation
Received : 06 October 2020
Accepted : 09 April 2021
Published : 08 June 2021
DOI : https://doi.org/10.1038/s41539-021-00089-5
Share this article
Anyone you share the following link with will be able to read this content:
Sorry, a shareable link is not currently available for this article.
Provided by the Springer Nature SharedIt content-sharing initiative
This article is cited by
Relation of life sciences students’ metacognitive monitoring to neural activity during biology error detection.
- Mei Grace Behrendt
- Carrie Clark
- Joseph Dauer
npj Science of Learning (2024)
Inferential theories of retrospective confidence
- Bennett L. Schwartz
Metacognition and Learning (2024)
Ambient smart environments: affordances, allostasis, and wellbeing
- Mark Miller
Synthese (2024)
The many facets of metacognition: comparing multiple measures of metacognition in healthy individuals
- Anneke Terneusen
- Conny Quaedflieg
- Ieke Winkens
Towards a common conceptual space for metacognition in perception and memory
- Audrey Mazancieux
- Michael Pereira
- Céline Souchay
Nature Reviews Psychology (2023)
Quick links
- Explore articles by subject
- Guide to authors
- Editorial policies
Sign up for the Nature Briefing newsletter — what matters in science, free to your inbox daily.

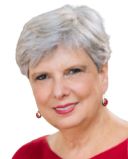
What Is Metacognition? How Does It Help Us Think?
Metacognitive strategies like self-reflection empower students for a lifetime..
Posted October 9, 2020 | Reviewed by Abigail Fagan
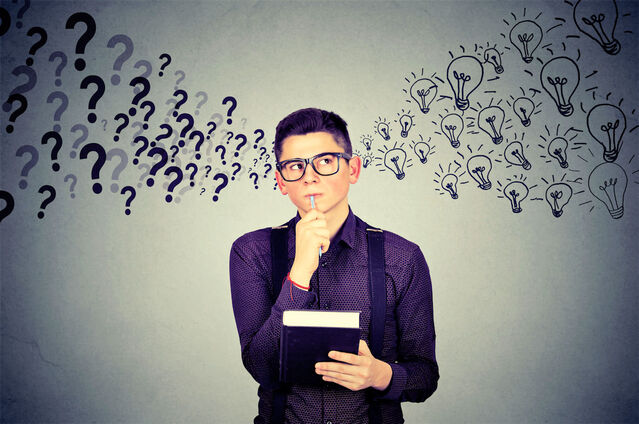
Metacognition is a high order thinking skill that is emerging from the shadows of academia to take its rightful place in classrooms around the world. As online classrooms extend into homes, this is an important time for parents and teachers to understand metacognition and how metacognitive strategies affect learning. These skills enable children to become better thinkers and decision-makers.
Metacognition: The Neglected Skill Set for Empowering Students is a new research-based book by educational consultants Dr. Robin Fogarty and Brian Pete that not only gets to the heart of why metacognition is important but gives teachers and parents insightful strategies for teaching metacognition to children from kindergarten through high school. This article summarizes several concepts from their book and shares three of their thirty strategies to strengthen metacognition.
What Is Metacognition?
Metacognition is the practice of being aware of one’s own thinking. Some scholars refer to it as “thinking about thinking.” Fogarty and Pete give a great everyday example of metacognition:
Think about the last time you reached the bottom of a page and thought to yourself, “I’m not sure what I just read.” Your brain just became aware of something you did not know, so instinctively you might reread the last sentence or rescan the paragraphs of the page. Maybe you will read the page again. In whatever ways you decide to capture the missing information, this momentary awareness of knowing what you know or do not know is called metacognition.
When we notice ourselves having an inner dialogue about our thinking and it prompts us to evaluate our learning or problem-solving processes, we are experiencing metacognition at work. This skill helps us think better, make sound decisions, and solve problems more effectively. In fact, research suggests that as a young person’s metacognitive abilities increase, they achieve at higher levels.
Fogarty and Pete outline three aspects of metacognition that are vital for children to learn: planning, monitoring, and evaluation. They convincingly argue that metacognition is best when it is infused in teaching strategies rather than taught directly. The key is to encourage students to explore and question their own metacognitive strategies in ways that become spontaneous and seemingly unconscious .
Metacognitive skills provide a basis for broader, psychological self-awareness , including how children gain a deeper understanding of themselves and the world around them.
Metacognitive Strategies to Use at Home or School
Fogarty and Pete successfully demystify metacognition and provide simple ways teachers and parents can strengthen children’s abilities to use these higher-order thinking skills. Below is a summary of metacognitive strategies from the three areas of planning, monitoring, and evaluation.
1. Planning Strategies
As students learn to plan, they learn to anticipate the strengths and weaknesses of their ideas. Planning strategies used to strengthen metacognition help students scrutinize plans at a time when they can most easily be changed.
One of ten metacognitive strategies outlined in the book is called “Inking Your Thinking.” It is a simple writing log that requires students to reflect on a lesson they are about to begin. Sample starters may include: “I predict…” “A question I have is…” or “A picture I have of this is…”
Writing logs are also helpful in the middle or end of assignments. For example, “The homework problem that puzzles me is…” “The way I will solve this problem is to…” or “I’m choosing this strategy because…”
2. Monitoring Strategies
Monitoring strategies used to strengthen metacognition help students check their progress and review their thinking at various stages. Different from scrutinizing, this strategy is reflective in nature. It also allows for adjustments while the plan, activity, or assignment is in motion. Monitoring strategies encourage recovery of learning, as in the example cited above when we are reading a book and notice that we forgot what we just read. We can recover our memory by scanning or re-reading.
One of many metacognitive strategies shared by Fogarty and Pete, called the “Alarm Clock,” is used to recover or rethink an idea once the student realizes something is amiss. The idea is to develop internal signals that sound an alarm. This signal prompts the student to recover a thought, rework a math problem, or capture an idea in a chart or picture. Metacognitive reflection involves thinking about “What I did,” then reviewing the pluses and minuses of one’s action. Finally, it means asking, “What other thoughts do I have” moving forward?

Teachers can easily build monitoring strategies into student assignments. Parents can reinforce these strategies too. Remember, the idea is not to tell children what they did correctly or incorrectly. Rather, help children monitor and think about their own learning. These are formative skills that last a lifetime.
3. Evaluation Strategies
According to Fogarty and Pete, the evaluation strategies of metacognition “are much like the mirror in a powder compact. Both serve to magnify the image, allow for careful scrutiny, and provide an up-close and personal view. When one opens the compact and looks in the mirror, only a small portion of the face is reflected back, but that particular part is magnified so that every nuance, every flaw, and every bump is blatantly in view.” Having this enlarged view makes inspection much easier.
When students inspect parts of their work, they learn about the nuances of their thinking processes. They learn to refine their work. They grow in their ability to apply their learning to new situations. “Connecting Elephants” is one of many metacognitive strategies to help students self-evaluate and apply their learning.
In this exercise, the metaphor of three imaginary elephants is used. The elephants are walking together in a circle, connected by the trunk and tail of another elephant. The three elephants represent three vital questions: 1) What is the big idea? 2) How does this connect to other big ideas? 3) How can I use this big idea? Using the image of a “big idea” helps students magnify and synthesize their learning. It encourages them to think about big ways their learning can be applied to new situations.
Metacognition and Self-Reflection
Reflective thinking is at the heart of metacognition. In today’s world of constant chatter, technology and reflective thinking can be at odds. In fact, mobile devices can prevent young people from seeing what is right before their eyes.
John Dewey, a renowned psychologist and education reformer, claimed that experiences alone were not enough. What is critical is an ability to perceive and then weave meaning from the threads of our experiences.
The function of metacognition and self-reflection is to make meaning. The creation of meaning is at the heart of what it means to be human.
Everyone can help foster self-reflection in young people.
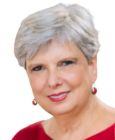
Marilyn Price-Mitchell, Ph.D., is an Institute for Social Innovation Fellow at Fielding Graduate University and author of Tomorrow’s Change Makers.
- Find a Therapist
- Find a Treatment Center
- Find a Psychiatrist
- Find a Support Group
- Find Online Therapy
- United States
- Brooklyn, NY
- Chicago, IL
- Houston, TX
- Los Angeles, CA
- New York, NY
- Portland, OR
- San Diego, CA
- San Francisco, CA
- Seattle, WA
- Washington, DC
- Asperger's
- Bipolar Disorder
- Chronic Pain
- Eating Disorders
- Passive Aggression
- Personality
- Goal Setting
- Positive Psychology
- Stopping Smoking
- Low Sexual Desire
- Relationships
- Child Development
- Self Tests NEW
- Therapy Center
- Diagnosis Dictionary
- Types of Therapy
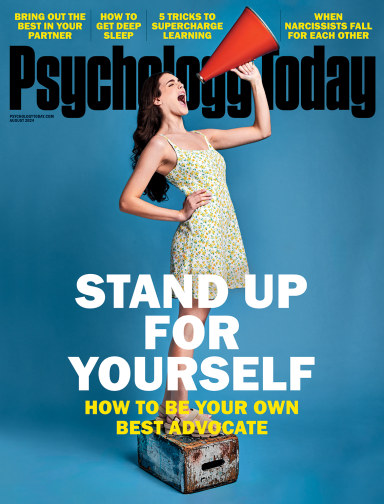
Sticking up for yourself is no easy task. But there are concrete skills you can use to hone your assertiveness and advocate for yourself.
- Emotional Intelligence
- Gaslighting
- Affective Forecasting
- Neuroscience
Center for Teaching
Metacognition.
Chick, N. (2013). Metacognition. Vanderbilt University Center for Teaching. Retrieved [todaysdate] from https://cft.vanderbilt.edu/guides-sub-pages/metacognition/. |
Thinking about One’s Thinking | Putting Metacognition into Practice
Thinking about One’s Thinking
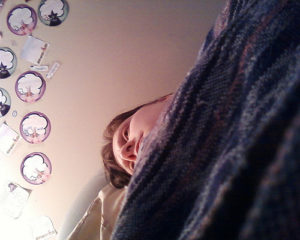
Initially studied for its development in young children (Baker & Brown, 1984; Flavell, 1985), researchers soon began to look at how experts display metacognitive thinking and how, then, these thought processes can be taught to novices to improve their learning (Hatano & Inagaki, 1986). In How People Learn , the National Academy of Sciences’ synthesis of decades of research on the science of learning, one of the three key findings of this work is the effectiveness of a “‘metacognitive’ approach to instruction” (Bransford, Brown, & Cocking, 2000, p. 18).
Metacognitive practices increase students’ abilities to transfer or adapt their learning to new contexts and tasks (Bransford, Brown, & Cocking, p. 12; Palincsar & Brown, 1984; Scardamalia et al., 1984; Schoenfeld, 1983, 1985, 1991). They do this by gaining a level of awareness above the subject matter : they also think about the tasks and contexts of different learning situations and themselves as learners in these different contexts. When Pintrich (2002) asserts that “Students who know about the different kinds of strategies for learning, thinking, and problem solving will be more likely to use them” (p. 222), notice the students must “know about” these strategies, not just practice them. As Zohar and David (2009) explain, there must be a “ conscious meta-strategic level of H[igher] O[rder] T[hinking]” (p. 179).
Metacognitive practices help students become aware of their strengths and weaknesses as learners, writers, readers, test-takers, group members, etc. A key element is recognizing the limit of one’s knowledge or ability and then figuring out how to expand that knowledge or extend the ability. Those who know their strengths and weaknesses in these areas will be more likely to “actively monitor their learning strategies and resources and assess their readiness for particular tasks and performances” (Bransford, Brown, & Cocking, p. 67).
The absence of metacognition connects to the research by Dunning, Johnson, Ehrlinger, and Kruger on “Why People Fail to Recognize Their Own Incompetence” (2003). They found that “people tend to be blissfully unaware of their incompetence,” lacking “insight about deficiencies in their intellectual and social skills.” They identified this pattern across domains—from test-taking, writing grammatically, thinking logically, to recognizing humor, to hunters’ knowledge about firearms and medical lab technicians’ knowledge of medical terminology and problem-solving skills (p. 83-84). In short, “if people lack the skills to produce correct answers, they are also cursed with an inability to know when their answers, or anyone else’s, are right or wrong” (p. 85). This research suggests that increased metacognitive abilities—to learn specific (and correct) skills, how to recognize them, and how to practice them—is needed in many contexts.
Putting Metacognition into Practice
In “ Promoting Student Metacognition ,” Tanner (2012) offers a handful of specific activities for biology classes, but they can be adapted to any discipline. She first describes four assignments for explicit instruction (p. 116):
- Preassessments—Encouraging Students to Examine Their Current Thinking: “What do I already know about this topic that could guide my learning?”
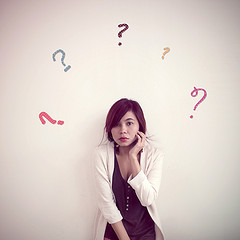
- Retrospective Postassessments—Pushing Students to Recognize Conceptual Change: “Before this course, I thought evolution was… Now I think that evolution is ….” or “How is my thinking changing (or not changing) over time?”
- Reflective Journals—Providing a Forum in Which Students Monitor Their Own Thinking: “What about my exam preparation worked well that I should remember to do next time? What did not work so well that I should not do next time or that I should change?”
Next are recommendations for developing a “classroom culture grounded in metacognition” (p. 116-118):
- Giving Students License to Identify Confusions within the Classroom Culture: ask students what they find confusing, acknowledge the difficulties
- Integrating Reflection into Credited Course Work: integrate short reflection (oral or written) that ask students what they found challenging or what questions arose during an assignment/exam/project
- Metacognitive Modeling by the Instructor for Students: model the thinking processes involved in your field and sought in your course by being explicit about “how you start, how you decide what to do first and then next, how you check your work, how you know when you are done” (p. 118)
To facilitate these activities, she also offers three useful tables:
- Questions for students to ask themselves as they plan, monitor, and evaluate their thinking within four learning contexts—in class, assignments, quizzes/exams, and the course as a whole (p. 115)
- Prompts for integrating metacognition into discussions of pairs during clicker activities, assignments, and quiz or exam preparation (p. 117)
- Questions to help faculty metacognitively assess their own teaching (p. 119)
Weimer’s “ Deep Learning vs. Surface Learning: Getting Students to Understand the Difference ” (2012) offers additional recommendations for developing students’ metacognitive awareness and improvement of their study skills:
“[I]t is terribly important that in explicit and concerted ways we make students aware of themselves as learners. We must regularly ask, not only ‘What are you learning?’ but ‘How are you learning?’ We must confront them with the effectiveness (more often ineffectiveness) of their approaches. We must offer alternatives and then challenge students to test the efficacy of those approaches. ” (emphasis added)
She points to a tool developed by Stanger-Hall (2012, p. 297) for her students to identify their study strategies, which she divided into “ cognitively passive ” (“I previewed the reading before class,” “I came to class,” “I read the assigned text,” “I highlighted the text,” et al) and “ cognitively active study behaviors ” (“I asked myself: ‘How does it work?’ and ‘Why does it work this way?’” “I wrote my own study questions,” “I fit all the facts into a bigger picture,” “I closed my notes and tested how much I remembered,” et al) . The specific focus of Stanger-Hall’s study is tangential to this discussion, 1 but imagine giving students lists like hers adapted to your course and then, after a major assignment, having students discuss which ones worked and which types of behaviors led to higher grades. Even further, follow Lovett’s advice (2013) by assigning “exam wrappers,” which include students reflecting on their previous exam-preparation strategies, assessing those strategies and then looking ahead to the next exam, and writing an action plan for a revised approach to studying. A common assignment in English composition courses is the self-assessment essay in which students apply course criteria to articulate their strengths and weaknesses within single papers or over the course of the semester. These activities can be adapted to assignments other than exams or essays, such as projects, speeches, discussions, and the like.
As these examples illustrate, for students to become more metacognitive, they must be taught the concept and its language explicitly (Pintrich, 2002; Tanner, 2012), though not in a content-delivery model (simply a reading or a lecture) and not in one lesson. Instead, the explicit instruction should be “designed according to a knowledge construction approach,” or students need to recognize, assess, and connect new skills to old ones, “and it needs to take place over an extended period of time” (Zohar & David, p. 187). This kind of explicit instruction will help students expand or replace existing learning strategies with new and more effective ones, give students a way to talk about learning and thinking, compare strategies with their classmates’ and make more informed choices, and render learning “less opaque to students, rather than being something that happens mysteriously or that some students ‘get’ and learn and others struggle and don’t learn” (Pintrich, 2002, p. 223).
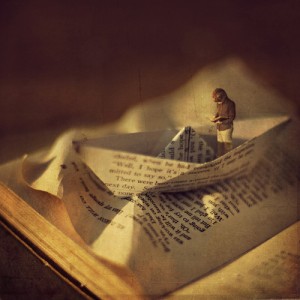
- What to Expect (when reading philosophy)
- The Ultimate Goal (of reading philosophy)
- Basic Good Reading Behaviors
- Important Background Information, or discipline- and course-specific reading practices, such as “reading for enlightenment” rather than information, and “problem-based classes” rather than historical or figure-based classes
- A Three-Part Reading Process (pre-reading, understanding, and evaluating)
- Flagging, or annotating the reading
- Linear vs. Dialogical Writing (Philosophical writing is rarely straightforward but instead “a monologue that contains a dialogue” [p. 365].)
What would such a handout look like for your discipline?
Students can even be metacognitively prepared (and then prepare themselves) for the overarching learning experiences expected in specific contexts . Salvatori and Donahue’s The Elements (and Pleasures) of Difficulty (2004) encourages students to embrace difficult texts (and tasks) as part of deep learning, rather than an obstacle. Their “difficulty paper” assignment helps students reflect on and articulate the nature of the difficulty and work through their responses to it (p. 9). Similarly, in courses with sensitive subject matter, a different kind of learning occurs, one that involves complex emotional responses. In “ Learning from Their Own Learning: How Metacognitive and Meta-affective Reflections Enhance Learning in Race-Related Courses ” (Chick, Karis, & Kernahan, 2009), students were informed about the common reactions to learning about racial inequality (Helms, 1995; Adams, Bell, & Griffin, 1997; see student handout, Chick, Karis, & Kernahan, p. 23-24) and then regularly wrote about their cognitive and affective responses to specific racialized situations. The students with the most developed metacognitive and meta-affective practices at the end of the semester were able to “clear the obstacles and move away from” oversimplified thinking about race and racism ”to places of greater questioning, acknowledging the complexities of identity, and redefining the world in racial terms” (p. 14).
Ultimately, metacognition requires students to “externalize mental events” (Bransford, Brown, & Cocking, p. 67), such as what it means to learn, awareness of one’s strengths and weaknesses with specific skills or in a given learning context, plan what’s required to accomplish a specific learning goal or activity, identifying and correcting errors, and preparing ahead for learning processes.
————————
1 Students who were tested with short answer in addition to multiple-choice questions on their exams reported more cognitively active behaviors than those tested with just multiple-choice questions, and these active behaviors led to improved performance on the final exam.
- Adams, Maurianne, Bell, Lee Ann, and Griffin, Pat. (1997). Teaching for diversity and social justice: A sourcebook . New York: Routledge.
- Bransford, John D., Brown Ann L., and Cocking Rodney R. (2000). How people learn: Brain, mind, experience, and school . Washington, D.C.: National Academy Press.
- Baker, Linda, and Brown, Ann L. (1984). Metacognitive skills and reading. In Paul David Pearson, Michael L. Kamil, Rebecca Barr, & Peter Mosenthal (Eds.), Handbook of research in reading: Volume III (pp. 353–395). New York: Longman.
- Brown, Ann L. (1980). Metacognitive development and reading. In Rand J. Spiro, Bertram C. Bruce, and William F. Brewer, (Eds.), Theoretical issues in reading comprehension: Perspectives from cognitive psychology, linguistics, artificial intelligence, and education (pp. 453-482). Hillsdale, NJ: Erlbaum.
- Chick, Nancy, Karis, Terri, and Kernahan, Cyndi. (2009). Learning from their own learning: how metacognitive and meta-affective reflections enhance learning in race-related courses . International Journal for the Scholarship of Teaching and Learning, 3(1). 1-28.
- Commander, Nannette Evans, and Valeri-Gold, Marie. (2001). The learning portfolio: A valuable tool for increasing metacognitive awareness . The Learning Assistance Review, 6 (2), 5-18.
- Concepción, David. (2004). Reading philosophy with background knowledge and metacognition . Teaching Philosophy , 27 (4). 351-368.
- Dunning, David, Johnson, Kerri, Ehrlinger, Joyce, and Kruger, Justin. (2003) Why people fail to recognize their own incompetence . Current Directions in Psychological Science, 12 (3). 83-87.
- Flavell, John H. (1985). Cognitive development. Englewood Cliffs, NJ: Prentice Hall.
- Hatano, Giyoo and Inagaki, Kayoko. (1986). Two courses of expertise. In Harold Stevenson, Azuma, Horishi, and Hakuta, Kinji (Eds.), Child development and education in Japan, New York: W.H. Freeman.
- Helms, Janet E. (1995). An update of Helms’ white and people of color racial identity models . In J.G. Ponterotto, Joseph G., Casas, Manuel, Suzuki, Lisa A., and Alexander, Charlene M. (Eds.), Handbook of multicultural counseling (pp. 181-198) . Thousand Oaks, CA: Sage.
- Lovett, Marsha C. (2013). Make exams worth more than the grade. In Matthew Kaplan, Naomi Silver, Danielle LaVague-Manty, and Deborah Meizlish (Eds.), Using reflection and metacognition to improve student learning: Across the disciplines, across the academy . Sterling, VA: Stylus.
- Palincsar, Annemarie Sullivan, and Brown, Ann L. (1984). Reciprocal teaching of comprehension-fostering and comprehension-monitoring activities . Cognition and Instruction, 1 (2). 117-175.
- Pintrich, Paul R. (2002). The Role of metacognitive knowledge in learning, teaching, and assessing . Theory into Practice, 41 (4). 219-225.
- Salvatori, Mariolina Rizzi, and Donahue, Patricia. (2004). The Elements (and pleasures) of difficulty . New York: Pearson-Longman.
- Scardamalia, Marlene, Bereiter, Carl, and Steinbach, Rosanne. (1984). Teachability of reflective processes in written composition . Cognitive Science , 8, 173-190.
- Schoenfeld, Alan H. (1991). On mathematics as sense making: An informal attack on the fortunate divorce of formal and informal mathematics. In James F. Voss, David N. Perkins, and Judith W. Segal (Eds.), Informal reasoning and education (pp. 311-344). Hillsdale, NJ: Erlbaum.
- Stanger-Hall, Kathrin F. (2012). Multiple-choice exams: An obstacle for higher-level thinking in introductory science classes . Cell Biology Education—Life Sciences Education, 11(3), 294-306.
- Tanner, Kimberly D. (2012). Promoting student metacognition . CBE—Life Sciences Education, 11, 113-120.
- Weimer, Maryellen. (2012, November 19). Deep learning vs. surface learning: Getting students to understand the difference . Retrieved from the Teaching Professor Blog from http://www.facultyfocus.com/articles/teaching-professor-blog/deep-learning-vs-surface-learning-getting-students-to-understand-the-difference/ .
- Zohar, Anat, and David, Adi Ben. (2009). Paving a clear path in a thick forest: a conceptual analysis of a metacognitive component . Metacognition Learning , 4 , 177-195.

Photo credit: wittygrittyinvisiblegirl via Compfight cc
Photo Credit: Helga Weber via Compfight cc
Photo Credit: fiddle oak via Compfight cc
Teaching Guides
- Online Course Development Resources
- Principles & Frameworks
- Pedagogies & Strategies
- Reflecting & Assessing
- Challenges & Opportunities
- Populations & Contexts
Quick Links
- Services for Departments and Schools
- Examples of Online Instructional Modules
- Resource Collection
- State Resources
Access Resources for State Adult Education Staff
- Topic Areas
- About the Collection
- Review Process
- Reviewer Biographies
- Federal Initiatives
- COVID-19 Support
- ADVANCE Integrated Education and Training (IET)
- IET Train-the Trainer Resources
- IET Resource Repository
- Program Design
- Collaboration and Industry Engagement
- Curriculum and Instruction
- Policy and Funding
- Program Management - Staffing -Organization Support
- Student Experience and Progress
- Adult Numeracy Instruction 2.0
- Advancing Innovation in Adult Education
- Bridge Practices
- Holistic Approach to Adult Ed
- Integrated Education and Training (IET) Practices
- Secondary Credentialing Practices
- Business-Adult Education Partnerships Toolkit
- Partnerships: Business Leaders
- Partnerships: Adult Education Providers
- Success Stories
- Digital Literacy Initiatives
- Digital Resilience in the American Workforce
- Landscape Scan
- Publications and Resources
- DRAW Professional Development Resources
- Employability Skills Framework
- Enhancing Access for Refugees and New Americans
- English Language Acquisition
- Internationally-Trained Professionals
- Rights and Responsibilities of Citizenship and Civic Participation
- Workforce Preparation Activities
- Workforce Training
- Integrated Education and Training in Corrections
- LINCS ESL PRO
- Integrating Digital Literacy into English Language Instruction
- Meeting the Language Needs of Today's English Language Learner
- Open Educational Resources (OER) for English Language Instruction
- Preparing English Learners for Work and Career Pathways
- Recommendations for Applying These Resources Successfully
- Moving Pathways Forward
- Career Pathways Exchange
- Power in Numbers
- Adult Learner Stories
- Meet Our Experts
- Newsletters
- Reentry Education Tool Kit
- Education Services
- Strategic Partnerships
- Sustainability
- Transition Processes
- Program Infrastructure
- SIA Resources and Professional Development
- Fulfilling the Instructional Shifts
- Observing in Classrooms
- SIA ELA/Literacy Videos
- SIA Math Videos
- SIA ELA Videos
- Conducting Curriculum Reviews
- Boosting English Learner Instruction
- Student Achievement in Reading
- TEAL Just Write! Guide
- Introduction
- Fact Sheet: Research-Based Writing Instruction
- Increase the Amount of Student Writing
- Fact Sheet: Adult Learning Theories
- Fact Sheet: Student-Centered Learning
- Set and Monitor Goals
- Fact Sheet: Self-Regulated Learning
- Fact Sheet: Metacognitive Processes
- Combine Sentences
- Teach Self-Regulated Strategy Development
- Fact Sheet: Self-Regulated Strategy Development
- Teach Summarization
- Make Use of Frames
- Provide Constructive Feedback
- Apply Universal Design for Learning
- Fact Sheet: Universal Design for Learning
- Check for Understanding
- Fact Sheet: Formative Assessment
- Differentiated Instruction
- Fact Sheet: Differentiated Instruction
- Gradual Release of Responsibility
- Join a Professional Learning Community
- Look at Student Work Regularly
- Fact Sheet: Effective Lesson Planning
- Use Technology Effectively
- Fact Sheet: Technology-Supported Writing Instruction
- Project Resources
- Summer Institute
- Teacher Effectiveness in Adult Education
- Adult Education Teacher Induction Toolkit
- Adult Education Teacher Competencies
- Teacher Effectiveness Online Courses
- Teaching Skills that Matter
- Teaching Skills that Matter Toolkit Overview
- Teaching Skills that Matter Civics Education
- Teaching Skills that Matter Digital Literacy
- Teaching Skills that Matter Financial Literacy
- Teaching Skills that Matter Health Literacy
- Teaching Skills that Matter Workforce Preparation
- Teaching Skills that Matter Other Tools and Resources
- Technology-Based Coaching in Adult Education
- Technical Assistance and Professional Development
- About LINCS
- History of LINCS
- LINCS Guide
- Style Guide
TEAL Center Fact Sheet No. 4: Metacognitive Processes
Metacognition is one’s ability to use prior knowledge to plan a strategy for approaching a learning task, take necessary steps to problem solve, reflect on and evaluate results, and modify one’s approach as needed. It helps learners choose the right cognitive tool for the task and plays a critical role in successful learning.
What Is Metacognition?
Metacognition refers to awareness of one’s own knowledge—what one does and doesn’t know—and one’s ability to understand, control, and manipulate one’s cognitive processes (Meichenbaum, 1985). It includes knowing when and where to use particular strategies for learning and problem solving as well as how and why to use specific strategies. Metacognition is the ability to use prior knowledge to plan a strategy for approaching a learning task, take necessary steps to problem solve, reflect on and evaluate results, and modify one’s approach as needed. Flavell (1976), who first used the term, offers the following example: I am engaging in Metacognition if I notice that I am having more trouble learning A than B; if it strikes me that I should double check C before accepting it as fact (p. 232).
Cognitive strategies are the basic mental abilities we use to think, study, and learn (e.g., recalling information from memory, analyzing sounds and images, making associations between or comparing/contrasting different pieces of information, and making inferences or interpreting text). They help an individual achieve a particular goal, such as comprehending text or solving a math problem, and they can be individually identified and measured. In contrast, metacognitive strategies are used to ensure that an overarching learning goal is being or has been reached. Examples of metacognitive activities include planning how to approach a learning task, using appropriate skills and strategies to solve a problem, monitoring one’s own comprehension of text, self-assessing and self-correcting in response to the self-assessment, evaluating progress toward the completion of a task, and becoming aware of distracting stimuli.
Elements of Metacognition
Researchers distinguish between metacognitive knowledge and metacognitive regulation (Flavell, 1979, 1987; Schraw & Dennison, 1994). Metacognitive knowledge refers to what individuals know about themselves as cognitive processors, about different approaches that can be used for learning and problem solving, and about the demands of a particular learning task. Metacognitive regulation refers to adjustments individuals make to their processes to help control their learning, such as planning, information management strategies, comprehension monitoring, de-bugging strategies, and evaluation of progress and goals. Flavell (1979) further divides metacognitive knowledge into three categories:
- Person variables: What one recognizes about his or her strengths and weaknesses in learning and processing information.
- Task variables: What one knows or can figure out about the nature of a task and the processing demands required to complete the task—for example, knowledge that it will take more time to read, comprehend, and remember a technical article than it will a similar-length passage from a novel.
- Strategy variables: The strategies a person has “at the ready” to apply in a flexible way to successfully accomplish a task; for example, knowing how to activate prior knowledge before reading a technical article, using a glossary to look up unfamiliar words, or recognizing that sometimes one has to reread a paragraph several times before it makes sense.
Livingston (1997) provides an example of all three variables: “I know that I ( person variable ) have difficulty with word problems ( task variable ), so I will answer the computational problems first and save the word problems for last ( strategy variable ).”
Why Teach Metacognitive Skills?
Research shows that metacognitive skills can be taught to students to improve their learning (Nietfeld & Shraw, 2002; Thiede, Anderson, & Therriault, 2003).
Constructing understanding requires both cognitive and metacognitive elements. Learners “construct knowledge” using cognitive strategies, and they guide, regulate, and evaluate their learning using metacognitive strategies. It is through this “thinking about thinking,” this use of metacognitive strategies, that real learning occurs. As students become more skilled at using metacognitive strategies, they gain confidence and become more independent as learners.
Individuals with well-developed metacognitive skills can think through a problem or approach a learning task, select appropriate strategies, and make decisions about a course of action to resolve the problem or successfully perform the task. They often think about their own thinking processes, taking time to think about and learn from mistakes or inaccuracies (North Central Regional Educational Laboratory, 1995). Some instructional programs encourage students to engage in “metacognitive conversations” with themselves so that they can “talk” with themselves about their learning, the challenges they encounter, and the ways in which they can self-correct and continue learning.
Moreover, individuals who demonstrate a wide variety of metacognitive skills perform better on exams and complete work more efficiently—they use the right tool for the job, and they modify learning strategies as needed, identifying blocks to learning and changing tools or strategies to ensure goal attainment. Because Metacognition plays a critical role in successful learning, it is imperative that instructors help learners develop metacognitively.
What’s the Research?
Metacognitive strategies can be taught (Halpern, 1996), they are associated with successful learning (Borkowski, Carr, & Pressley, 1987). Successful learners have a repertoire of strategies to select from and can transfer them to new settings (Pressley, Borkowski, & Schneider, 1987). Instructors need to set tasks at an appropriate level of difficulty (i.e., challenging enough so that students need to apply metacognitive strategies to monitor success but not so challenging that students become overwhelmed or frustrated), and instructors need to prompt learners to think about what they are doing as they complete these tasks (Biemiller & Meichenbaum, 1992). Instructors should take care not to do the thinking for learners or tell them what to do because this runs the risk of making students experts at seeking help rather than experts at thinking about and directing their own learning. Instead, effective instructors continually prompt learners, asking “What should you do next?”
McKeachie (1988) found that few college instructors explicitly teach strategies for monitoring learning. They assume that students have already learned these strategies in high school. But many have not and are unaware of the metacognitive process and its importance to learning. Rote memorization is the usual—and often the only—learning strategy employed by high school students when they enter college (Nist, 1993). Simpson and Nist (2000), in a review of the literature on strategic learning, emphasize that instructors need to provide explicit instruction on the use of study strategies. The implication for ABE programs is that it is likely that ABE learners need explicit instruction in both cognitive and metacognitive strategies. They need to know that they have choices about the strategies they can employ in different contexts, and they need to monitor their use of and success with these strategies.
Recommended Instructional Strategies
Instructors can encourage ABE learners to become more strategic thinkers by helping them focus on the ways they process information. Self-questioning, reflective journal writing, and discussing their thought processes with other learners are among the ways that teachers can encourage learners to examine and develop their metacognitive processes.
Fogarty (1994) suggests that Metacognition is a process that spans three distinct phases, and that, to be successful thinkers, students must do the following:
- Develop a plan before approaching a learning task, such as reading for comprehension or solving a math problem.
- Monitor their understanding; use “fix-up” strategies when meaning breaks down.
- Evaluate their thinking after completing the task.
Instructors can model the application of questions, and they can prompt learners to ask themselves questions during each phase. They can incorporate into lesson plans opportunities for learners to practice using these questions during learning tasks, as illustratetd in the following examples:
- During the planning phase, learners can ask, What am I supposed to learn? What prior knowledge will help me with this task? What should I do first? What should I look for in this reading? How much time do I have to complete this? In what direction do I want my thinking to take me?
- During the monitoring phase, learners can ask, How am I doing? Am I on the right track? How should I proceed? What information is important to remember? Should I move in a different direction? Should I adjust the pace because of the difficulty? What can I do if I do not understand?
- During the evaluation phase, learners can ask, H ow well did I do? What did I learn? Did I get the results I expected? What could I have done differently? Can I apply this way of thinking to other problems or situations? Is there anything I don’t understand—any gaps in my knowledge? Do I need to go back through the task to fill in any gaps in understanding? How might I apply this line of thinking to other problems?
Rather than viewing reading, writing, science, social studies, and math only as subjects or content to be taught, instructors can see them as opportunities for learners to reflect on their learning processes. Examples follow for each content area:
- Reading: Teach learners how to ask questions during reading and model “think-alouds.” Ask learners questions during read-alouds and teach them to monitor their reading by constantly asking themselves if they understand what the text is about. Teach them to take notes or highlight important details, asking themselves, “Why is this a key phrase to highlight?” and “Why am I not highlighting this?”
- Writing: Model prewriting strategies for organizing thoughts, such as brainstorming ideas using a word web, or using a graphic organizer to put ideas into paragraphs, with the main idea at the top and the supporting details below it.
- Social Studies and Science: Teach learners the importance of using organizers such as KWL charts, Venn diagrams, concept maps , and anticipation/reaction charts to sort information and help them learn and understand content. Learners can use organizers prior to a task to focus their attention on what they already know and identify what they want to learn. They can use a Venn diagram to identify similarities and differences between two related concepts.
- Math: Teach learners to use mnemonics to recall steps in a process, such as the order of mathematical operations. Model your thought processes in solving problems—for example, “This is a lot of information; where should I start? Now that I know____, is there something else I know?”
The goal of teaching metacognitive strategies is to help learners become comfortable with these strategies so that they employ them automatically to learning tasks, focusing their attention, deriving meaning, and making adjustments if something goes wrong. They do not think about these skills while performing them but, if asked what they are doing, they can usually accurately describe their metacognitive processes.
Biemiller, A., & Meichenbaum, D. (1992). The nature and nurture of the self-directed learner. Educational Leadership, 50, 75–80.
Borkowski, J., Carr, M., & Pressely, M. (1987). “Spontaneous” strategy use: Perspectives from metacognitive theory. Intelligence, 11, 61–75.
Flavell, J. H. (1979). Metacognition and cognitive monitoring: A new area of cognitive-developmental inquiry. American Psychologist, 34, 906–911.
Flavell, J. H. (1976). Metacognitive aspects of problem solving. In L. B. Resnick (Ed.), The nature of intelligence (pp. 231–236). Hillsdale, NJ: Lawrence Erlbaum Associates.
Flavell, J. H. (1987). Speculations about the nature and development of metacognition. In F. E. Weinert & R. H. Kluwe (Eds.), Metacognition, motivation, and understanding (pp. 21–29). Hillside, NJ: Lawrence Erlbaum Associates.
Fogarty, R. (1994). How to teach for metacognition. Palatine, IL: IRI/Skylight Publishing.
Halpern, D. F. (1996). Thought and knowledge: An introduction to critical thinking. Mahwah, NJ: Lawrence Erlbaum Associates.
Livingston, J. A. (1997). Metacognition: An overview. Retrieved December 27, 2011 from http://gse.buffalo.edu/fas/shuell/CEP564/Metacog.htm
McKeachie, W. J. (1988). The need for study strategy training. In C. E. Weinstein, E. T. Goetz, & P. A. Alexander (Eds.), Learning and study strategies: Issues in assessment, instruction, and evaluation (pp. 3–9). New York: Academic Press.
Meichenbaum, D. (1985). Teaching thinking: A cognitive-behavioral perspective. In S. F., Chipman, J. W. Segal, & R. Glaser (Eds.), Thinking and learning skills, Vol. 2: Research and open questions. Hillsdale, NJ: Lawrence Erlbaum Associates.
North Central Regional Educational Laboratory. (1995). Strategic teaching and reading project guidebook. Retrieved December 27, 2011
Nietfeld, J. L., & Shraw, G. (2002). The effect of knowledge and strategy explanation on monitoring accuracy. Journal of Educational Research, 95, 131–142.
Nist, S. (1993). What the literature says about academic literacy. Georgia Journal of Reading, Fall-Winter, 11–18.
Pressley, M., Borkowski, J. G., & Schneider, W. (1987). Cognitive strategies: Good strategy users coordinate metacognition and knowledge. In R. Vasta, & G. Whitehurst (Eds.), Annals of child development, 4, 80–129. Greenwich, CT: JAI Press.
Schraw, G., & Dennison, R. S. (1994). Assessing metacognitive awareness. Contemporary Educational Psychology, 19, 460–475.
Simpson, M. L., & Nist, S. L. (2000). An update on strategic learning: It’s more than textbook reading strategies. Journal of Adolescent and Adult Literacy, 43 (6) 528–541.
Thiede, K. W., Anderson, M. C., & Therriault, D. (2003). Accuracy of metacognitive monitoring affects learning of texts. Journal of Educational Psychology, 95, 66–73.
Authors: TEAL Center staff
Reviewed by: David Scanlon, Boston College
About the TEAL Center: The Teaching Excellence in Adult Literacy (TEAL) Center is a project of the U.S. Department of Education, Office of Career, Technical, and Adult Education (OCTAE), designed to improve the quality of teaching in adult education in the content areas.

Maintenance work is planned from 21:00 BST on Tuesday 20th August 2024 to 21:00 BST on Wednesday 21st August 2024, and on Thursday 29th August 2024 from 11:00 to 12:00 BST.
During this time the performance of our website may be affected - searches may run slowly, some pages may be temporarily unavailable, and you may be unable to log in or to access content. If this happens, please try refreshing your web browser or try waiting two to three minutes before trying again.
We apologise for any inconvenience this might cause and thank you for your patience.
Chemistry Education Research and Practice
Metacognitive problem solving: exploration of students’ perspectives through the lens of multi-dimensional engagement.
Solving chemical problems entails content knowledge and mastery of problem-solving processes. However, students sometimes lack metacognitive processes required for problem solving in chemistry. This study investigated how first-year chemistry students engaged with the metacognitive problem-solving scaffold Goldilocks Help. Data was collected from an activity, which involved students reflectively comparing their problem-solving attempts to an expert solution. These comparative reflections (N = 373) were thematically analysed to investigate scaffold engagement in three dimensions: cognitive, emotional, and behavioural. Finding showed that scaffold use, coupled with self-reflection, allowed students to identify flaws in their solutions that were either problem specific or related to their problem-solving skills. Students were able to propose improvement strategies, such as posing prompting questions to themselves and finding multiple alternatives for evaluating an answer. Students, who initially lacked structured problem-solving skills, found that scaffolding helped them to slow down metacognitive processes that would otherwise be rushed through or engaged with on a surface level. Students’ resistance to the scaffold was due to fear of making a mistake or viewing the scaffold as requiring extra time and effort. Within a semester, many students demonstrated an improvement in successful and structured problem solving but some required more practice to internalise the scaffold. Our findings also indicated that students’ reflections on problem solving became more sophisticated as a result of continued exposure to the scaffold and iterative opportunities to compare their work to expert solutions, to self-assess, and to reflect. Further research on reflective writing in chemistry education should focus on the ipsative nature of such assessments, i.e. processes focussing on students’ own progress, growth, and improvement, compared to their previous performance, while recognising the power relations operationalised in course-embedded reflections. From the teaching practice perspective, having an awareness of students’ thoughts, emotions, and actions can help instructors differentiate between levels of student capabilities, mindsets, and needs for extra support, allowing teaching efforts to be directed at promoting metacognitive and structured problem solving.
Supplementary files
- Supplementary information PDF (125K)
Article information
Download citation, permissions.

K. Vo, M. Sarkar, P. J. White and E. Yuriev, Chem. Educ. Res. Pract. , 2024, Accepted Manuscript , DOI: 10.1039/D4RP00096J
To request permission to reproduce material from this article, please go to the Copyright Clearance Center request page .
If you are an author contributing to an RSC publication, you do not need to request permission provided correct acknowledgement is given.
If you are the author of this article, you do not need to request permission to reproduce figures and diagrams provided correct acknowledgement is given. If you want to reproduce the whole article in a third-party publication (excluding your thesis/dissertation for which permission is not required) please go to the Copyright Clearance Center request page .
Read more about how to correctly acknowledge RSC content .
Social activity
Search articles by author.
This article has not yet been cited.
Advertisements

An official website of the United States government
The .gov means it’s official. Federal government websites often end in .gov or .mil. Before sharing sensitive information, make sure you’re on a federal government site.
The site is secure. The https:// ensures that you are connecting to the official website and that any information you provide is encrypted and transmitted securely.
- Publications
- Account settings
Preview improvements coming to the PMC website in October 2024. Learn More or Try it out now .
- Advanced Search
- Journal List
- Am J Pharm Educ
- v.81(4); 2017 May
Strategies for Improving Learner Metacognition in Health Professional Education
Melissa s. medina.
a University of Oklahoma Health Sciences Center, Oklahoma City, Oklahoma
Ashley N. Castleberry
b University of Arkansas for Medical Sciences College of Pharmacy, Little Rock, Arkansas

Adam M. Persky
c Eshelman School of Pharmacy, University of North Carolina at Chapel Hill, Chapel Hill, North Carolina
Metacognition is an essential skill in critical thinking and self-regulated, lifelong learning. It is important for learners to have skills in metacognition because they are used to monitor and regulate reasoning, comprehension, and problem-solving, which are fundamental components/outcomes of pharmacy curricula. Instructors can help learners develop metacognitive skills within the classroom and experiential setting by carefully designing learning activities within courses and the curriculum. These skills are developed through intentional questioning, modeling techniques, and reflection. This article discusses key background literature on metacognition and identifies specific methods and strategies to develop learners’ metacognitive skills in both the classroom and experiential settings.
INTRODUCTION
Imagine the following situation: You ask your class to find and review a journal article. One of your learners, Morgan, begins the assignment the night before the assignment due date. She is unsure where to search for primary literature. Once she finds an article, she underestimates the review time. She spends several hours reviewing and finishes the assignment in the early morning. When the instructor graded the assignment, she receives a poor grade because most questions were not directly and concisely answered. Morgan is surprised by her grade because she spent several hours working on the assignment, which was more than she thought would be necessary.
While many things could explain Morgan’s behavior, at the foundation Morgan may have trouble with metacognition. She failed to plan. She did not know where to look for information. She misjudged time. She did not check her work for accuracy. She was overconfident in her predicted grade. All these elements point to poor metacognitive skills.
What is Metacognition?
We can define metacognition as the “thinking about thinking.” Because it refers to a person’s “knowledge and cognition about cognitive phenomena.” 1,2 This type of cognition regulates thinking and learning and consists of three self-assessment skills: planning, monitoring, and evaluating. In the case of Morgan, she failed to lay out a plan for her article review; during the process, did not monitor whether she was accomplishing the goal; and once done, did not evaluate her work for correctness.
Researchers have investigated three aspects of metacognition: metacognitive knowledge, metacognitive monitoring, and metacognitive control. 3 Metacognitive knowledge is the information you consult when thinking about an idea; it includes the basic facts and concepts. Metacognitive monitoring is the ability to assess cognitive activity whereas metacognitive control is the ability to regulate cognitive activity. In the example above, Morgan may have lacked the knowledge of where to look for an article or how to review an article (metacognitive knowledge). She may have lacked the ability to assess whether she was answering the relevant questions (metacognitive monitoring). She also may be deficient in the control of metacognition by allowing insufficient time for this activity (metacognitive control).
Importance of Metacognition
Metacognition is important to every profession. There are many reasons why metacognition is important in the health sciences, including from being a better learner to becoming a better clinician. During the learning process, metacognition guides our learning strategies. If learners know what they know and do not know, they can focus on acquiring the knowledge they are lacking. Metacognitive skills also have a role in critical thinking and problem solving. If you know what you know and do not know, your metacognitive skills help drive you to obtain the missing information, which we refer to as self-directed or self-regulated learning. Finally, being mindful or metacognitively aware can prevent medication errors in clinical settings because of increases in awareness of our thought process leading to better critical thinking and monitoring of actions. As an example, self-assessment errors routinely occur among physicians, nurses, pharmacists and other health care providers. 4
Metacognition in Medical Errors
Medical errors are one of the leading causes of death in many countries. 5 Researchers have argued that medical error is partly a cognitive issue. 6 Medication order entry errors were the fourth leading cause of medication errors in 2003. 7 These types of errors can occur because the pharmacist did not ask “do the orders make sense for the indication?” (ie, metacognitive monitoring) or “did I check to ensure I entered things correctly?” (ie, evaluation of medical orders or evaluation of entered data). One study cited that most medication errors occur at the prescription and found 40% of errors were related to prescribing the wrong dose and 18% from omitted information. 8
In addition, physicians, pharmacists, and other health care providers can be overconfident in their assessments leading to medical errors which can lead to hindsight bias which may further hinder learning. Hindsight bias is the “knew it all along” effect and is the belief that an event is more predictable after it becomes known than it was before it became known. During hindsight bias, we lose the ability to recollect the feeling of uncertainty that preceded an event. This bias hinders our appraisal of past events.
Part of the cause of hindsight bias is the subjective feeling of ease associated when we make judgments – a metacognitive function. When people find it easy to come to a conclusion about a particular outcome, they will show greater hindsight bias, particularly regarding foreseeability (“I knew that would happen.”). One reason is that people attribute the subjective ease of the judgment to the certainty of being correct – the answer came easily and thus must be correct. In one study, physicians were asked to guess the likelihood that they would get the correct diagnosis in the future (prospective or foresight) and in the past (retrospectively or hindsight). 9 The less experienced physicians gave significantly higher estimates in hindsight than in foresight. For easier cases, the more experienced physicians demonstrated hindsight bias; however, for more difficult cases they did not give higher estimates than in foresight. In another study, physicians were asked their confidence and accuracy during right heart catheterization. 10 Physicians were confident of their estimates, but there was no relationship between confidence and accuracy. Experienced physicians were no more accurate than less experienced ones, although they were significantly more confident. These are two examples of how metacognitive skills may affect clinical judgments.
Metacognition and Study Skills
Metacognition is crucial in controlling and guiding thinking. 1 Dunlosky proposed a model of how metacognitive control impacts study time. 11 This model included study preparation (self-efficacy evaluation, task appraisal, and initial strategy selection) followed by monitoring and assessing whether the to-be-learned items has been learned and feeding back into the cycle to re-study unlearned material. Several investigators found that learners are unaware of effective study strategies which impact performance. 12-14
College learners have displayed overconfidence in self-chosen study strategies about academic performance and have demonstrated low correlations between self-predicted and actual performance on learning assessments. 15,16 This pattern of overconfidence may be more apparent in low-performing learners. 17,18 The issue of selecting study strategies is complicated because a requirement for selecting a learning strategy is metacognitive knowledge about which learning strategies are beneficial for long-term memory. Several studies report learners using low-impact study strategies such as rereading or highlighting notes. 12,19,20 In one study, 80% of undergraduates reported that the study skills they use were learned on their own and not taught to them in a formal manner by teachers. 16 These findings are consistent with the health science literature that also found rereading a prominent learning strategy. 20,21 The selection of poor study strategies raises questions of whether those improvised strategies, presumably based on intuition or metacognitive feedback, are consistent with the evidence. This hypothesis raises a second question whether instruction on learning and memory topics could improve metacognitive awareness of successful learning strategies. Recently it was documented that learners who have engaged in their study skills development use better strategies, but low impact strategies still predominate. 20 As a result, faculty members are advised to teach study skills formally to learners. 22
Metacognition and Self-Directed Learning
Most health-professional organizations and accrediting bodies encourage lifelong learning because of the ever-changing biomedical landscape. Lifelong learning requires self-direction and self-regulation. Self-directed learning is the result of allowing learners to make decisions about the information they want to experience or learn. 23 In a realistic learning situation, self-directed learning is difficult and in a formal education setting, information selection is limited and governed by the instructor. 24 While this is limiting, instructors need to set learning objectives for novice learners because these learners are not in a position to be self-directed. They do not know the skills and knowledge needed to become a health professional; also, limiting resources to find information may be appropriate early on to help build efficiency into the learning process (ie, learners do not have to spend large amounts of time searching for relevant information).
Providing guidance can lead to a “passive learning environment.” However, within a passive learning environment, learners selectively attend to different environmental cues. 25 As an example of a passive environment, learners actively evaluate what has been said, or engage in self-explanation to decide what other information is required. 24 Self-directed learning differs from self-regulation. For example, if the article Morgan found was interesting, she could be self-directed in learning more about the topic. However, she would be self-regulated when balancing this additional learning with her required course learning.
Self-regulation is how individuals guide their goal-directed activities over time. It is designed to maximize the long-term best interests of an individual, resulting in learners controlling their impulses and looking out for their well-being. 26 Self-regulated learning modulates various processes (eg, cognitive, behavioral) to reach the desired goal. These regulatory mechanisms are the essentials of self-regulated learning because they are under the control of the learners and would be the basis for future professional development (ie, continual professional development). The self-regulated behaviors include planning, monitoring, attention, and effort.
Both planning and monitoring are components of metacognition. When learners engage in planning activities, they think through what they need to learn and set task-specific goals. 27,28 Once they plan, they need to monitor. Monitoring refers to paying attention to one’s performance and understanding of the course material. 29 Monitoring is a critical component of self-regulation because it provides awareness of one’s knowledge level, which then leads to changes in one’s affect, cognition, and behavior. 27 Accurate monitoring enhances the regulation of learning because it provides feedback to what trainees already know and where they need to focus their resources. 28,30
Metacognition and Critical Thinking
Critical thinking involves cognitive, dispositional and metacognitive components. The cognitive component represents the abilities to comprehend a problem and apply cognitive skills to make sound judgments. The disposition component influences the patterns of intellectual activity; these can include the enjoyment of thinking, an open attitude, a careful approach and a mindset for truth seeking. 31,32 Metacognitive strategies enable learners to supervise and control their thinking processes. At its core, a critical thinker is one in charge of their thinking processes, while metacognitive strategies enable such control to take place. The metacognitive aspects interact with a variety of internal and external factors like type of instruction, motivation, and socio-economic status ( Figure 1 ).

Influence of Internal and External Factors in Cognition
Metacognition is the ability to monitor thinking to use skills and strategies appropriately to achieve a desirable outcome. We discussed this regarding learning strategies and parallels can be made for critical thinking skills. Halpern defines a critical thinker as one who applies appropriate skills and strategies to achieve a desirable outcome. 33 As such, critical thinkers strategically use cognitive skills that are best suited to a particular situation. They are aware of their thinking and thus control their thinking processes. Metacognitive strategies are an important variable during thinking processes. 33 These skills need to be made explicit and public to develop critical thinking skills. 33
Metacognition and the Accreditation Process
Accreditation standards for pharmacy (ACPE) and medicine (AAMC) emphasize metacognitive skills as a critical part of health professional training. For pharmacy education, the college or school provides an environment and culture that promotes self-awareness, self-directed lifelong learning, professional behavior, leadership, collegial relationships, and collaboration within and across academic units, disciplines, and professions. (Standards 4 and 9). The experiential curriculum should provide an inculcation of habits of self-directed lifelong learning (Standard 12). Standard 4 explicitly states self-awareness as a key factor and that learners use metacognitive practice to regulate their learning. The appendices further emphasize self-directed and lifelong learning including continuous professional development. Similarly, in medicine we see an emphasis on self-directed learning. The faculty of a medical school should ensure that the medical curriculum includes self-directed learning experiences and time for independent study to allow medical learners to develop the skills of lifelong learning. Self-directed learning involves medical learners’ self-assessment of learning needs; independent identification, analysis, and synthesis of relevant information; and appraisal of the credibility of information sources (Standard 6.3).
Overview of Instructional Approaches to Teach Metacognition
Metacognitive processes are best taught in conjunction and alignment with cognitive processes and separating the two processes is challenging. 34 One key instructional strategy in developing metacognition is cognitive apprenticeship. Cognitive apprenticeship is “learning through guided experience.” In this model, the expert’s cognitive and metacognitive processes and skills used when performing a task are explicit and public and are the focus of teaching activities. 35 There are four dimensions to this model that reflect the three dimensions of metacognition.
The first dimension includes content knowledge (concepts, facts, procedures) and strategic knowledge (heuristic, metacognitive, learning). To think through a process, one must have the content knowledge to think about something. One also needs to have a heuristic (short-cut) or algorithm (formula) to follow to develop the skill. Thus, instruction should have a content component and direct instruction on how to work through a process.
The second dimension of cognitive apprenticeship has a method to demonstrate metacognition. The expert or instructor should model the process – externalizing the thought process. The concept of coaching is appropriate as the expert should help demonstrate and coach learners through the process. Within this procedure, learners reflect on their thoughts, verbalizing their motives and assumptions. 34 The process should be scaffolded, ie, offering early examples or demonstrations in a more supportive model leading to a level of independence.
The third dimension of cognitive apprenticeship is an extension of scaffolding, and this concept is the importance of sequencing: increasing task complexity, diversity of problems, and migrating from global to local skills. Task complexity starts with straightforward problems and building complexity with experience. The diversity of problems helps learners build more generalizable knowledge and skills. When we use a diversity of problems, we are approaching similar problems from different contexts to help build context-independent knowledge and skills. When we learn, we form a memory trace for that information. This memory is dependent on the cues available during the learning context. 36 We can recall this information only if we receive the correct cues. If we see the content or skill (or thought process) with a wide array of problems, we can retrieve the information from a variety of cues and contexts and start to make generalities. Finally, after faculty scaffold and sequence appropriately, they move the learners from global skills to local skills. At this point, learners should have a clear conceptual model of the task or process before executing its parts. Developing global skills reflects the idea that seeing the overall structure of the problem or content helps in understanding the individual parts because we can draw on relationships to help reinforce the learning. 37
The fourth dimension of cognitive apprenticeship is the sociology of learning and includes situated learning, a community of practice, intrinsic motivation, and exploiting cooperation. This idea is consistent with factors of motivation especially relatedness – we are more motivated to learn or perform when we can relate to the situation or the person. The authenticity of the learning environment (experiential vs. classroom) or the problem (patient case vs. foundational science) helps frame the real-world context which increases motivation. Therefore, situated learning (environment reflects the real-world) increases motivation. 38-40 The last components are social in nature but also consistent with effective learning strategies: a community of practice (engaging in a community to achieve goals) and cooperation (cooperation between learners in problem solving). These methods are real-world since health care is a team process and the research consistently show that learners teaching other learners is an effective strategy (effect sizes above 0.70). 41 Cooperative learning allows for a variety of positive attributes including feedback and communication which help in the metacognitive process.
Strategies to Enhance Metacognition in the Classroom
Several methods can be used to enhance learners’ metacognition in the classroom. Methods used during any part of normal instructional approaches include lecture, active learning exercises, or pre-planned activities outside of the classroom. Example methods can be modified based on the knowledge level of the learner and number of learners in the classroom in combination with scaffolding. To note, developing metacognition within learners is not an easy task. 34 Appendix 1 contains some sample metacognitive learning objectives.
General Planning
Learners plan better and learn when their attention focuses on learning objectives established by the instructor. The explicit discussion of the learning objectives starts the metacognitive process by prioritizing the importance of thinking about the learning process over the content. To activate prior knowledge, prompt the learners to think about what they already know that is related to the content of that day and what relevant knowledge they lack. 42 Next, lead the learners to analyze the distinctions between contrasting information and focus more on these differences rather than the similarity between concepts. 43,44 Have learners assess the time it will take to complete this activity and where they will find the resources for successful completion of the task to help them think about the process of studying. Additional self-questions to promote learner metacognition about learning can be found in Table 1 . 45
Sample Self-Questions to Promote Learner Metacognition About Learning 45,66-68

General Monitoring
Learners benefit from monitoring their understanding (ie, metacognitive awareness) during teaching activities. 46-48 By checking learning behavior throughout the lecture or teaching activity, learners are reminded of the importance of the learning process. Learners can accomplish this by noting important concepts and writing down questions during the lecture or activity. 45 Jotting down questions can be facilitated by dividing content into 10-15 minute segments and offering activities to refocus attention to the learning objectives and reflecting on their comprehension of the material. The instructor can help learners learn strategies for retaining information such as chunking, connecting, and elaborating and assist them in organizing the material in ways to recognize patterns and associations. They may regulate the difficulty of the material by breaking down the problem into simpler steps for learners to clearly see the thought process of problem-solving. 43 After some practice with simpler questions, incrementally increase the difficulty of the problem. Another way to assist learners in monitoring their thought processes is to provide half-done examples and have learners solve them then discuss possible conclusions. By monitoring smaller pieces of an assignment, the instructor and learners are better able to identify and correct errors in thinking. ( Table 1 ).
General Evaluating
By evaluating metacognitive skills, learners become more aware of this process and its impact on learning. Creating checklists, rating scales, and rubrics for distribution before the assignment can help learners monitor and evaluate their thinking as they are working. Additionally, administering a metacognitive questionnaire during an exam can help learners evaluate their thinking during the exam and make corrections accordingly ( Appendix 2 provides an example). 49 Reviewing this questionnaire after the exam along with individual results can also be helpful to identify patterns of incorrect thinking or gaps in preparation and study time. Having learners evaluate their learning is powerful and can lead to change for future learning ( Table 1 ).
Other Strategies Examination Reviews
Examinations themselves can be a metacognitive method. 50,51 Reviewing an examination with learners after grades are released can be a powerful way to help them start thinking about their thought process during the examination. Examination reviews occur in a group setting with the entire class or during a one-on-one or small group interaction with learners. During this session, have learners reflect on their answer choice and the actual correct answer. Ask them to reflect on why they got the item incorrect and specifically why this occurred. Ask prompting questions such as: What were your assumptions about this item? What are some alternative ways to approach this question? What piece of knowledge were you missing? By forcing learners to identify the problem in their thought process for each item, you might be able to discern a pattern in behaviors and offer strategies to remedy this. In addition, the small delay in feedback also can enhance learning. 52
In addition to reviewing the exam after administration and scoring, a review before the exam can help learners assess their learning strategies and adjust accordingly before the exam. Doing an exam review a couple of days before the exam still gives learners time to change their studying and enhance weak areas. By using questions similar to the format of an examination question, learners familiarize themselves with the examination requirements. Using active learning strategies paired with a group discussion on questions that require critical thinking can be a powerful review tool for learners. A review before and after an examination can benefit learner learning.
Thinking out Loud
A form of modeling is thinking out loud, and this occurs in the classroom. 43 For example, provide learners with a complex question or case scenario and let them think about their approach to solving the issue. Then think out loud to model your thought process for how you would solve this issue as a content expert. Have learners compare their thought process to yours to identify gaps, errors, or alignment to improve their thinking. This method also benefits learners in experiential training such as clinical rotations or shadowing experiences. Expert thinking is often internal and not portrayed to learner learners who can negatively reinforce wrong thinking. For example, when experts are problem-solving, they silently sort relevant and irrelevant information before generating a solution. To a novice learner, all of the information can appear important, which can hinder their problem solving. Experts should instead think out loud so novices can better understand what is irrelevant in certain problem-solving situations. By thinking out loud, you show learners how to approach situations and model the process of monitoring your behaviors in professional practice.
Reflection is simply the intentional and dynamic process that allows improvement in one’s actions, abilities, and knowledge by learning from past experiences. 53 To reflect, think back on an experience and analyze the situation. By getting learners to reflect, they think about their actions, abilities, and knowledge and assess improvement in these areas moving forward. Reflection assignments following learning activities (whether in the classroom, simulation, or practice) can help learners think about their thinking and develop plans to grow in these areas. Reflective writing assignments can include responses to three questions: What worked well when preparing for this exam/quiz/assignment? What did not work well when preparing for this exam/quiz/assignment? What will I change before my next exam/quiz/assignment? 45
Reflection using the “Muddiest Point” allows learners to identify confusion during a lecture or learning activity. 45 Have learners write down what part of the material remains confusing to them. Then have the learners investigate the issue further to encourage exploration of knowledge and self-directed learning. This quick exercise can have a high yield for metacognitive practice.
Another reflective method is self-explanation. When learners use self-explanation, they are asking themselves to explain their process and what they can do next time. This technique has been used to facilitate the transfer of learning and problem solving. 54
Adding Judgments of Understanding
Asking learners to prospectively make judgments (eg, I predict my score on this exam to be…) or retrospectively make judgments (eg, for the test question I just completed, my confidence in my answer is…) can help learners monitor or evaluate their learning. These types of judgments have been used within higher education and courses in pharmacy. 17,18,55 When asked these questions, learner accuracy in predicting grades improves and moves from being overconfident to underconfident with reductions in bias. 18,49 See Appendix 3 for an example of a weekly monitoring exercise with judgments of understanding.
Strategies to Enhance Metacognition in the Experiential Setting
In addition to classroom instruction, raising learners’ metacognition is important in the experiential setting. To date, there is less research in this educational setting, but opportunities exist to develop and research metacognitive development.
Mastery Goal Setting
Artino and colleagues in 2012 found that learners’ metacognitive skills (planning, goal setting, monitoring comprehension, and evaluating learning) correlated positively with mastery goal structures, which are environments that emphasize developing competence, mastering new skills and learning to understand. 56 Mastery goal structures contrast with performance-approach goal structures that focus on demonstrating proficiency and peer comparison and performance-avoid goal structures that encourage avoiding looking incompetent. 56 Both performance-approach and avoidance structures are associated with procrastination, avoidance of help-seeking, and poor grades, which are goal orientations that become predominant in the experiential setting. 56 Learners in the experiential setting may resort to these goal orientations because it is a time when the bulk of learners’ grades are derived directly from observation instead of tests, and they may adapt behaviors to avoid looking incompetent. 56 Based on these findings, preceptors should consider encouraging learners to adapt mastery-oriented goals and seek help when needed. One way preceptors support mastery goal structures is to offer formative assessments paired with feedback that emphasizes progress and mastery of knowledge, skills, and attitudes. 56 These formative assessments can take a variety of forms from case discussions, journal clubs, or presentations – typically practices that normally occur during experiential rotations. These should regularly occur to help the learner develop. These feedback sessions may be an opportunity to use the verbalization strategies found in Appendix 4 .
Questioning and Feedback
Another way preceptors can help their learners on rotation is to emphasize metacognitive skills from the beginning of training coupled with immediate feedback regarding technique. This method was shown to help novice medical learners on a surgery rotation learn laparoscopic surgery skills using simulation software. 57 There are questions that preceptors regularly can ask learners to promote metacognitive awareness, including questions related to planning, monitoring, and evaluating. Research with elementary school learners showed that when teachers asked learners two questions, “what did you learn about yourself today regarding the subject area?” and “What did you learn that you can consistently replicate well?” their metacognitive awareness increased. 58 Based on these results, preceptors could ask learners questions found in Table 2 .
Questions to Ask During Experiential Settings

Another important aspect of experiential education is the purpose of asking questions. Often we might hear of preceptors “pimping” learners – that is to ask questions under the guise of educating them but the real purpose is to determine hierarchy. Questioning should be Socratic and developmental compared to evaluative and demeaning ( Table 3 ). 59-62
Comparison of the Socratic Teaching Method vs Clinical “Pimping”

To effectively ask questions, preceptors should do the following: 59 diagnose the learners (ie, what level are they at) and teach to that level; avoid asking questions for questions’ sake (for eg, questions about trivia, historical facts, non-meaningful eponyms, and impossible, guess-what-I’m-thinking questions); tell learners your goal in asking questions; emphasize important learning points; and do not attempt to embarrass intentionally or humiliate the learners.
Clinical Documentation with Explanation
Written and verbal communication require an explanation of the thought process. For example, if you are making a recommendation to a physician for a specific drug, you should explain why you chose that drug (drug allergy to another drug, lab levels, adverse effects, other disease states). By explicitly documenting reasoning, metacognition is modeled and could lead to better communication and patient outcomes. As an example, SOAP notes may include an additional page that documents the rationalization and resources used to arrive at the plan. SOAP notes have been used to improve reflection and learning in medical physiology in medical learners and help faculty reflect on learning in dental hygiene education. 63 The use of clinical documentation may be especially impactful when novices can compare their documents to experienced clinicians and when clinicians verbalize their process as they develop the document.
Experiential Rotation Structure
Finally, the overall structure of experiential education should be scaffolded – both between and within a rotation. Learners should be started with a more supportive environment and moved toward more independence; this can support their metacognitive development. This scaffolding or progressive problem-solving approach is a critical part of developing expertise. It is the gaining of experience for both content but process that is important. Experts are more metacognitively aware than novices because the progression of problem solving requires the cognitive and metacognitive processes. 64
Metacognition refers to a person’s ability to regulate their thinking and learning and consists of the self-assessment skills: planning, monitoring, and evaluating. These important skills reduce self-assessment errors, such as hindsight bias, among health care providers. The new pharmacy, medical, and nursing education accreditation standards emphasize metacognitive skills and the related skills in critical thinking and self-directed learning. Studies reporting formal teaching of these skills are often lacking which further emphasizes the need to teach health care learners explicitly metacognitive skills during their training. Suggestions for teaching metacognitive skills in the didactic setting include cognitive apprenticeships, exam reviews, modeling of metacognitive skills, thinking out loud protocols, reflection assignments, self-explanation methods, and judgment of understanding assignments. In the experiential setting, faculty members can emphasize mastery goal setting, use questioning techniques that promote metacognitive awareness coupled with feedback about learner efforts in this area, request clinical documentation with an explanation, and scaffold learners during the rotation. Overall, using these teaching strategies regardless of setting can raise learners’ self-awareness and help metacognitive thinking to occur more automatically. Metacognition’s role in clinical decision making is important as it is a means to address “what to learn,” “when to learn,” and “how to learn.” 65
ACKNOWLEDGMENTS
The authors thank Cindy Stowe and John Dunlosky for their input during manuscript preparation, and Kayley Lyons, Shelby Hudson, and Tom Angelo for their help during the editing process.
Appendix 1. Outcome/Objective Statements
By the end of this course, you should be able to:
Comprehend the limits of your memory for a particular task and create a means of external support.
Self-monitor your learning strategies and then adapt the strategies if they are effective.
Notice whether you comprehend something you just read and then modify your approach if you did not comprehend it.
Skim subheadings of unimportant information to get to the information you need.
Rehearse a skill to gain proficiency.
Self-test to see how well you learned something.
Verbalize your thought process for a particular task.
Appendix 2. Exam Item Assessment

Appendix 3. Example Weekly Monitoring Exercise

Appendix 4. Example of Verbalization Techniques to Develop Metacognition

Identifying Metacognitive Behavior in Problem-Posing Processes
Development of a Framework and a Proof of Concept
- Open access
- Published: 05 September 2022
- Volume 21 , pages 1381–1406, ( 2023 )
Cite this article
You have full access to this open access article
- Lukas Baumanns ORCID: orcid.org/0000-0002-6697-3994 1 &
- Benjamin Rott ORCID: orcid.org/0000-0002-8113-1584 1
4231 Accesses
7 Citations
1 Altmetric
Explore all metrics
Insights into the process of mathematical problem posing is a central concern in mathematics education research. However, little is known about regulative or metacognitive behaviors that are essential to understanding this process. In this study, we investigate metacognitive behavior in problem posing. We aim at (1) identifying problem-posing-specific metacognitive behaviors and (2) applying these identified metacognitive behaviors to illustrate differences in problem-posing processes. For these aims, we identified problem-posing-specific metacognitive behaviors of planning , monitoring & control , and evaluating in task-based interviews with primary and secondary pre-service teachers. As a proof of concept, the identified behaviors are applied on two selected transcript fragments to illustrate how a problem-posing-specific framework of metacognitive behavior reveals differences in problem-posing processes.
Similar content being viewed by others
Making Mathematics Challenging Through Problem Posing in the Classroom
A Review on Problem Posing in Teacher Education
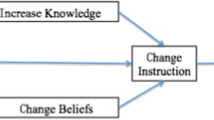
Learning to teach mathematics through problem posing: teachers’ beliefs and performance on problem posing
Avoid common mistakes on your manuscript.
Introduction
In research on problem posing, analyzing the products, that is the posed problems, plays an important role. One reason for this is that the ability to pose problems is often assessed by the posed problems (Bonotto, 2013 ; Singer et al., 2017 ; Van Harpen & Sriraman, 2013 ). However, in our analyses of problem-posing processes, we found that the observable quality of processes can differ, even though the same problems are posed, as will be illustrated with two exemplary processes. One construct that has made this difference within processes tangible for us is metacognitive behavior .
At least since Flavell’s ( 1979 ) seminal work, metacognition has been a central construct of research in psychology and is an established topic of mathematics education research (Schneider & Artelt, 2010 ). In particular, research on problem solving has benefited from the consideration of metacognitive behavior in the past decades. There are numerous characterizations of metacognitive behavior in problem solving (Artzt & Armour-Thomas, 1992 ; Garofalo & Lester, 1985 ; Schoenfeld, 1987 ; Yimer & Ellerton, 2010 ) as well as studies on the connection between metacognitive behavior and successful problem solving (Desoete et al., 2001 ; Kuzle, 2013 ; Özsoy & Ataman, 2009 ; Rott, 2013 ).
Although problem-solving research has benefited greatly from this perspective, it is noteworthy that far less conceptual or empirical research has been conducted in the related field of problem posing. A systematic literature review in high-ranked mathematics education journals has revealed that there are only a few studies that explicitly investigate problem-posing-specific aspects of metacognitive behavior (Baumanns & Rott, 2021a ). For example, activities of reflection are observed where problem posers consider solvability or the appropriateness of the posed problem for a specific target group (Kontorovich et al., 2012 ; Pelczer & Gamboa, 2009 ). Furthermore, there is a lack of a framework that explicitly addresses the analysis of metacognitive behavior in problem-posing processes. That is in particular striking as researchers often note that the field of problem posing lacks conceptual insights into the activity. Such insights would enable a better analysis and interpretation of the activity itself (Ellerton et al., 2015 ; Ruthven, 2020 ; Van Harpen & Sriraman, 2013 ). Similar to research on problem solving, it can be assumed that the consideration and analysis of problem-posing-specific aspects of metacognitive behavior may be a central enrichment to the field. This can contribute to a better understanding of problem-posing processes.
Based on this research desideratum, this article aims at (1) identifying problem-posing-specific aspects of metacognitive behaviors in problem-posing processes and (2) applying these identified metacognitive behaviors to illustrate differences in problem-posing processes.
Theoretical Background
Problem posing.
Similar to problem solving, problem posing is considered to be a central activity of mathematicians’ (Hadamard, 1945 ; Halmos, 1980 ; Lang, 1989 ). Already (Pólya, 1945 ) mentioned problem posing as a partial activity in the context of problem solving. Despite this recognition by mathematicians, for a long time, problem posing has received noticeably less attention in mathematics education research than problem solving. At the latest since the 1980s (Brown & Walter, 1983 ; Butts, 1980 ; Kilpatrick, 1987 ), problem posing has been increasingly investigated. In recent years, researchers from mathematics education have shown an increasing interest in investigating and understanding problem posing (Cai et al., 2015 ; Cai & Hwang, 2020 ; Cai & Leikin, 2020 ; Lee, 2020 ; Silver, 2013 ). For example, problem posing has been widely used to identify or assess mathematical creativity (Joklitschke et al., 2019 ; Silver, 1997 ; Singer & Voica, 2015 ; Van Harpen & Sriraman, 2013; Yuan & Sriraman, 2011 ).
There are numerous definitions of problem posing that conceptualize more or less equivalent activities. Silver ( 1994 ) defines problem posing as the generation of new problems and reformulation of given problems which can occur before, during, or after a problem-solving process. Stoyanova and Ellerton, ( 1996 , p. 218) refer to problem posing as the “process by which, on the basis of mathematical experience, students construct personal interpretations of concrete situations and formulate them as meaningful mathematical problems.” Cai and Hwang ( 2020 , p. 2) subsume under problem posing “several related types of activity that entail or support teachers and students formulating (or reformulating) and expressing a problem or task based on a particular context.”
Based on the categories by Stoyanova and Ellerton ( 1996 ), we distinguish between unstructured and structured problem-posing situations depending on the degree of given information (Baumanns & Rott, 2021b ). Unstructured situations are characterized by a given naturalistic or constructed situation in which tasks can be posed without or with less restrictions, for example “Consider the following infinite sequence of digits: 123456789101112131415…999100010011002… Note that it is made by writing the base ten counting numbers in order. Ask some meaningful questions. Put them in a suitable order” (Stoyanova, 1999 , p. 32). To pose meaningful questions, the structure of the situation has to be explored using mathematical knowledge and concepts. In structured situations, people are asked to pose further problems based on a specific problem, for example by varying its conditions. As structured situations are used in this study, examples can be seen in Table 2 .
- Metacognition
Going back all the way to Flavell ( 1979 ), who significantly influenced early research on this topic, metacognition is described as “knowledge and cognition about cognitive phenomena,” which roughly means thinking about thinking . Based on this understanding, two facets of metacognition are distinguished: (1) knowledge about cognition (Cross & Paris, 1988 ; Kuhn & Dean, 2004 ; Pintrich, 2002 ) and (2) regulation of cognition (Schraw & Moshman, 1995 ; Whitebread et al., 2009 ).
Knowledge about cognition includes declarative knowledge of strategy, task, and person (Pintrich, 2002 ). Strategic knowledge refers to knowledge about strategies (e.g. when solving problems) and when to apply them. Knowledge of tasks refers to knowing about different degrees of difficulty of tasks and different strategies required to solve them, for example. Person’s knowledge includes knowledge about one’s own strengths and weaknesses (e.g. in problem solving)
Regulation of cognition refers to procedural knowledge with regard to processes that coordinate cognition. This facet includes the activities of planning , monitoring , control , and evaluating (Pintrich, 2000 ). Planning refers to setting of a target goal concerning the current endeavor, the activation of prior content knowledge, and activation of metacognitive knowledge, for example knowledge about specific tasks and how to solve them. Monitoring refers to metacognitive awareness and monitoring of cognition, for example when verifying that one has understood the current task. Control refers to the selection and adaption of strategies, for example with the goal to solve a problem. Evaluating refers to activities of reflecting and judging on one’s own performance and results in the form of an outcome. These activities are not completely distinct from each other and there are different perspectives on their overlap. We follow the perspective of Pintrich ( 2000 ), who states that control is mostly conceptualized as dependent or at least highly similar to monitoring (Pintrich, 2000 ). By that, we also follow the approach of Cohors-Fresenborg and Kaune ( 2007 ) who summarize monitoring and control under one category (see also Schraw & Moshman, 1995 ). This is also consistent with past work by Pólya ( 1945 ), who indicated roughly these three activities in problem solving even before the construct metacognition was established (Cohors-Fresenborg et al., 2010 )
There are attempts to identify the behavior of planning, monitoring & control, and evaluating in learning contexts (Kaune, 2006 ; Van der Stel et al., 2010 ). For example, Cohors-Fresenborg and Kaune ( 2007 ) provide a category system for classifying teachers’ and students’ metacognitive activities in class discussions. The main categories of planning, monitoring & control (which they refer to as monitoring ), and evaluating (which they refer to as reflecting ) are divided into several subcategories with different aspects. Table 1 summarizes the codes for the main categories of planning, monitoring, and evaluating. Footnote 1 These codes are used to identify metacognitive behavior by teachers and students through the analysis of verbal protocols of classroom interactions. In addition to metacognitive behavior, Cohors-Fresenborg and Kaune ( 2007 ) also consider (negative) discursivity in their coding scheme. Discursivity is understood as a culture in which the teacher and the students always refer to each other’s expressions, work out differences in approaches, and regulate their own understanding. Because it is an additional aspect besides metacognition, and it is specific for classroom interactions which is not analyzed in the study at hand, the aspect of discursivity will not be considered further. In the study at hand, the focus is on the process of problem posing and, therefore, investigates regulation of cognition. In particular, we want to differentiate the activities of planning , monitoring & control , and evaluating for problem posing by adapting the approach by Cohors-Fresenborg and Kaune ( 2007 ).
Metacognition is often considered in conjunction with motivation and beliefs. Zimmerman and Moylan ( 2009 ) state that proactive self-regulation depends on the presence of motivational beliefs. For example, the metacognitive activity of planning depends highly on aspects like the intrinsic interest into the current endeavor, self-efficacy perceptions, or learning goal orientation. These aspects are deeply interwoven with motivation. It follows that for a holistic view of learners’ effort, for example in problem solving, metacognitive processes should be considered in addition to motivational beliefs (Zimmerman & Moylan, 2009 ).
Research on Metacognition in Mathematics Education
In mathematics education research, metacognition is of immense importance (for an overview, see Schneider & Artelt, 2010 ). Assessing metacognitive behavior is often used to investigate the mathematical skills of participants (Mevarech & Fridkin, 2006 ; Van der Stel et al., 2010 ). For example, Van der Stel et al. ( 2010 ) found out that the quality of metacognitive behavior seems to be a predictor of the mathematical performance in the future by analyzing the regulation of cognition in thinking-aloud protocols of second- and third-year students. Most prominently, metacognition is considered in problem-solving research. Considerations on this are mainly based on the ideas of Pólya ( 1945 ). Especially Schoenfeld ( 1985b , 1987 , 1992 ) emphasizes the importance of control or self-regulation in problem solving. He observed that even when students have the content knowledge necessary to solve a problem, a lack of ability to keep track of what they are doing, that is metacognitive behavior, might lead to failure in solving a problem. His theoretical and empirical analyses initiated numerous studies on metacognition in mathematics education — including the present study.
Extensive discussion has been done on characterizing metacognitive behavior in problem solving (Artzt & Armour-Thomas, 1992 ; Garofalo & Lester, 1985 ; Schoenfeld, 1985b ; Yimer & Ellerton, 2010 ). For example, Artzt and Armour-Thomas ( 1992 ) and Schoenfeld ( 1985b ) both identify the analysis of the problem and planning the solution as predominantly metacognitive behavior. In the analysis, selecting an appropriate way to reformulate the given problem, for example in order to make it simpler, is referred to as metacognitive behavior. The phase of planning is also metacognitive by nature as it involves controlling and self-regulating the solving process (Garofalo & Lester, 1985 ). Building on that, several studies investigate the relationship between metacognitive activities and successful problem solving and come to the result that successful problem solving is mostly associated with the presence of metacognitive activities (Desoete et al., 2001 ; Kim et al., 2013 ; Kuzle, 2013 ; Özsoy & Ataman, 2009 ).
Research on Metacognition in Problem Posing
Due to the relevance of considering metacognitive behavior in problem solving, it can be assumed that the analysis of metacognitive behavior could be similarly relevant in problem posing. However, the potential in this area has not been sufficiently exploited to date. As some researchers interpret problem posing as a problem-solving activity (Kontorovich et al., 2012 ; Silver, 1995 ) and since there are several established frameworks of metacognitive behavior in problem solving as described above, it is a reasonable question whether there is even a need for a separate framework for metacognitive behavior in problem posing. We follow the perspective of previous studies (Baumanns & Rott, 2022 ; Cruz, 2006 ; Pelczer & Gamboa, 2009 ) that argue, based on observations of problem-posing processes, that there are characteristic differences between problem-posing processes and problem-solving processes in general. Therefore, also metacognitive processes involved in problem posing differ from those in problem solving. Cognitive and metacognitive processes involved in problem posing seem to be of their own nature for which metacognitive frameworks for problem solving can only serve as a stimulus. From this derives the interest addressed in this study to investigate these problem-posing-specific aspects of metacognitive behavior.
Problem posing includes different cognitive and metacognitive processes (Baumanns & Rott, 2022 ; Christou et al., 2005 ; Koichu & Kontorovich, 2013 ; Pelczer & Gamboa, 2009 ) which are indicated in the following informally: Problem posers analyze the given situation, examine which mathematical knowledge could be relevant for this, and possibly look for structures in the situation that may lead to an interesting problem. Then, problems are posed and suitable representations of them are sought. The task may then be solved and while solving, the posers may reflect on the difficulty of the task, its appropriateness for an intended target group, or the general interest in the solution. These activities are not limited to cognitive processes, but may also contain metacognitive behavior. Cognitive behavior in problem posing is, for example, applying knowledge of previous mathematical experiences to a given problem-posing situation to pose a problem. Metacognitive behavior would be, for example, to attack a problem in order to assess whether the problem is well-defined or solvable at all.
Theoretical considerations on problem posing implicitly contain some aspects of metacognition and metacognitive regulation in particular (Carrillo & Cruz, 2016 ; Ghasempour et al., 2013 ; Kontorovich et al., 2012 ; Pelczer & Gamboa, 2009 ; Singer & Voica, 2015 ), yet metacognition is rarely explicitly addressed as the systematic literature review on problem-posing studies by Baumanns and Rott ( 2022 ) has revealed. The following sections address the few studies that review has identified that implicitly or explicitly address metacognitive behavior.
Voica et al. ( 2020 ) mention that they found metacognitive behavior in their study with students as they were able to analyze and reflect on their own posed problems and thinking processes which helped them to become aware of their strengths. Other studies have also pointed to the lack of metacognitive activities from the participants (Crespo, 2003 ; Tichá & Hošpesová, 2013 ). For example, Crespo ( 2003 ) writes that four of her thirteen participants “posed [problems] without solving beforehand or deeply understanding the mathematics” and they “indicate unawareness of the mathematical potential and scope of [their] problem[s]” (p. 251). Crespo ( 2003 ) identifies unawareness in her participants in the sense of a lack of reflection. This can also be interpreted as a lack of metacognitive behavior. Erkan and Kar ( 2022 ) were able to observe metacognitive factors in pre-service mathematics teachers, but these factors varied depending on the problem-posing situation. Their participants considered the strengths and weaknesses of their mathematical knowledge in order to write mathematically valid problems.
Pelczer and Gamboa ( 2009 ) as well as Kontorovich et al. ( 2012 ) specify thoughts on reflection on the posed problems. In their descriptive process model, Pelczer and Gamboa ( 2009 ) state that in the phase of evaluation , expert problem posers assess their posed problems in terms of various aspects, for example, whether further modifications are needed. In the phase of final assessment , the process of posing a problem is reflected upon and the problem itself is evaluated, for example in terms of difficulty and one’s own interest in solving it. Similarly, the framework for handling the complexity of problem-posing processes in small groups by Kontorovich et al. ( 2012 ) integrates the facet of individual considerations of aptness . Consideration of aptness includes, for example, to what extent the problem poser is satisfied with the quality of the posed problem or whether the posed problem is appropriate for a specific group of solvers.
Research Objectives
As the state of research has shown, metacognition has not been an important factor in problem-posing research. Therefore, the aim of this study is to offer a focused perspective on metacognitive behavior in problem posing. This lack of conceptual and empirical insight constitutes a desideratum which leads us to the following research objective:
Development of a framework for identifying problem-posing-specific aspects of metacognitive behavior (i.e. planning , monitoring & control , and evaluating ) in pre-service teachers’ problem-posing processes
As a second research objective, we pursue a proof of concept to identify differences in problem-posing processes based on metacognition. The problem-posing-specific metacognitive behaviors developed in research objective (1) will be applied to selected transcript excerpts of two problem-posing processes. This proof of concept is intended to show to what extent the analysis of metacognitive behaviors in problem-posing processes with the framework developed in (1) makes differences regarding metacognitive behavior in problem-posing processes visible.
Application of the framework developed in (1) as a proof of concept to make differences regarding metacognitive behavior in problem-posing processes visible
Research Design for Data Collection
There are several ways to assess metacognitive behavior, for example through interviews, stimulated recall, or eye-movement registration (for an overview, see Veenman et al., 2006 ). A clear distinction exists between off-line methods which are carried out before or after the current endeavor (often via self-report questionnaires), and on-line methods which are carried out during the current endeavor. As on-line methods seem to be more predictive with regard to the learning performance (Veenman et al., 2006 ), we chose the approach of video-based content analysis on problem-posing processes of pairs of pre-service teacher students. This method is based on the commonly used assumption that we can make interpretive conclusions about participants’ cognition and metacognition from their verbal expressions working in small groups (Artzt & Armour-Thomas, 1997 ; Goos et al., 2002 ).
The interviews were conducted in pairs in the same room to create a natural communication situation and eliminate the constructed pressure to produce something mathematical for the researcher (Schoenfeld, 1985a , p. 178). Johnson and Johnson ( 1999 ) underline that cooperative learning groups such as pairs are “windows into students’ minds” (p. 213). For this reason, the interviewer avoided intervening in the interaction process. The participants were asked to speak aloud at any time during interview while posing new problems. The interviews were conducted with 64 pre-service primary and secondary mathematics teachers. Sixteen students were in the first bachelor semester, 22 in the fifth bachelor semester, and 26 in the third master semester. The students worked in pairs on one of two structured problem-posing situations, either (A) Nim game or (B) Number pyramid (see Table 2 ). In total, 15 processes of situation (A) and 17 processes of situation (B) that range from 9 to 25 min with an average length of 14.5 min have been recorded. The processes ended when no ideas for further problems emerged from the participants. In total, 7h 46min of video material was recorded. Four pairs of students each were in the same room under authentic university seminar conditions. A camera was positioned opposite the pairs in order to capture all of the participants’ actions. In order to accustom them to a natural communication in front of the camera, short puzzles were performed before solving the initial task and the consecutive problem posing.
Data Analysis — Assessment of Metacognitive Behavior
To answer research question (1), we conducted a qualitative content analysis (Mayring, 2000 ). The main categories of the metacognitive behavior of planning (P), monitoring & control (MC), and evaluating (E) stem from theoretical considerations on regulation of cognition presented above (Schraw & Moshman, 1995 ; Whitebread et al., 2009 ), and more specifically from Cohors-Fresenborg’s and Kaune’s ( 2007 ; see Table 1 ) considerations. Although this framework is developed for analyzing classroom interaction, it has been used successfully in paired problem-solving processes (Rott, 2014 ). However, because problem posing is a mathematical activity of its own kind in our observations (Baumanns & Rott, 2022 ), a problem-posing-specific approach was chosen for the present proof of concept. The individual problem-posing-specific characteristics were obtained data-driven through an inductive category development (Mayring, 2000 ). The category development had four steps: (1) As the unit of analysis, the statements of the participants on the videotaped problem-posing processes were used for the category development. To identify the statements of planning , monitoring & control , and evaluating , the 32 recorded problem-posing processes were analyzed as follows: For the category of planning, we identified participants’ statements of setting a target goal for the current problem-posing situation, activating prior mathematical knowledge that helped posing new problems, or activating metacognitive knowledge in the form of knowledge on how to pose new problems in general. For the category of monitoring & control, we identified participants’ statements of awareness and monitoring of cognition as well as selecting and adapting problem-posing strategies. For the category of evaluating, we identified participants’ statements of assessing their problem-posing process or their products, that is their posed problems (e.g. “This is a good problem because it is not too difficult and it is novel compared to the initial problem.”). (2) For each identified statement in step 1, a short description was obtained (e.g. evaluation of the posed problem based on specific characteristics ). Similar descriptions within the main categories planning , monitoring & control , and evaluating were then clustered into subcategories. (3) Afterwards, the developed subcategories were revised by reanalyzing seven problem-posing processes in which particularly many and different metacognitive behaviors were observed and in which participants expressed particularly many verbalizations regarding the interpretation of metacognitive behavior. This reanalysis was used to further refine the descriptions of the categories. (4) After the categories were specified, all the video material was reviewed again in order to draw attention to possible additional categories. No new categories were found in this last step. The quality of the coding was ensured through consensual validation in team discussions which is a common method of ensuring scientific quality in qualitative research (Flick, 2007 ).
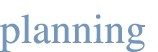
Development of a Framework for Identifying Problem-Posing-Specific Aspects of Metacognitive Behavior
We first want to establish the identified problem-posing-specific aspects of metacognitive behavior of planning , monitoring & control , and evaluating . To do that, the developed types of metacognitive behavior are described and anchor examples of observed processes with regard to the Nim game and Number pyramid (see Table 2 ) are presented.

Proof of Concept: the Cases of Tino & Ulrich and Valerie & Wenke
In this section, we will analyze two cases — one by Tino & Ulrich and the other by Valerie & Wenke — with regard to the aspects of metacognitive behavior that have been developed in the previous section. The aim of this section is to illustrate different degrees of metacognitive behavior in two problem-posing processes that have a similar product. The two selected fragments are identical in numerous features of the outer structure: All four participants are pre-service high-school teachers in their third semester of their master degree. In the analyzed fragments, they work on posing new problems for the Nim game (see Table 2 ). In the shown fragments, both pairs of participants pose the same problem, namely the variation of the Nim game in which the players are allowed to remove 2 or 3 stones from the table. The transcript analysis is conducted to illustrate the different degree of metacognitive behavior between those two processes in which a largely identical product is produced.
Tino & Ulrich — Analysis of the Metacognitive Behavior
The following excerpt from the process of Tino and Ulrich takes place in the first half of their process. Beforehand, they already posed, solved, and analyzed several new variations of the Nim game such as what if there are 21 stones on the table in the beginning? What if you could remove 1, 2, or 3 stones from the table? What if you also get a winning point if you have removed more stones from the table than your opponent? Then, they pose the problem that you are only allowed to remove 2 or 3 stones from the table. The development of this problem is shown in the following transcribed excerpt that takes 3m 12s. For a better visual assessment of the density of metacognitive behavior, in this transcript, the codes for the metacognitive behavior are already set and marked in color.
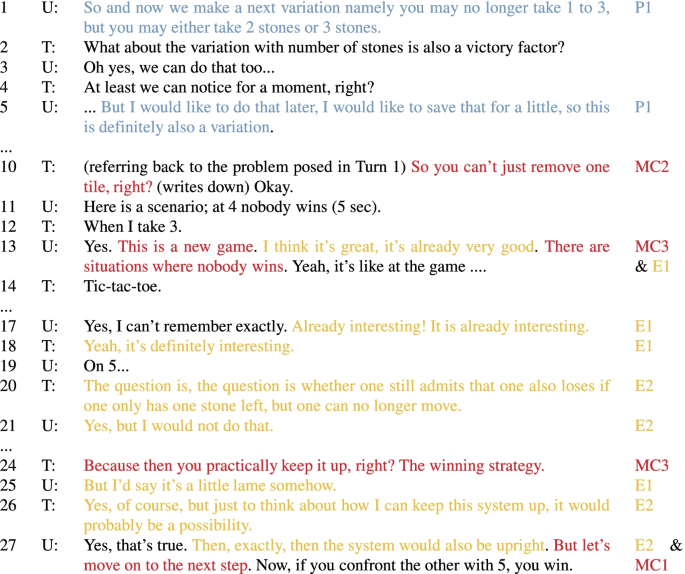
Valerie & Wenke — Analysis of the Metacognitive Behavior
The following excerpt from the process of Valerie and Wenke takes place quite early in the process. Beforehand, they both analyzed the solution to the initial problem and then asked themselves how they could now come to new problems or what they could specifically vary about the game in order to come to new problems. Afterwards, they pose the variation that you may only remove 2 or 3 stones from the table. The following excerpt takes 1m 28s and shows the creation of this problem. As in the section before, the codes for the metacognitive behavior are already set and marked in color. The coding is commented on after the transcript.
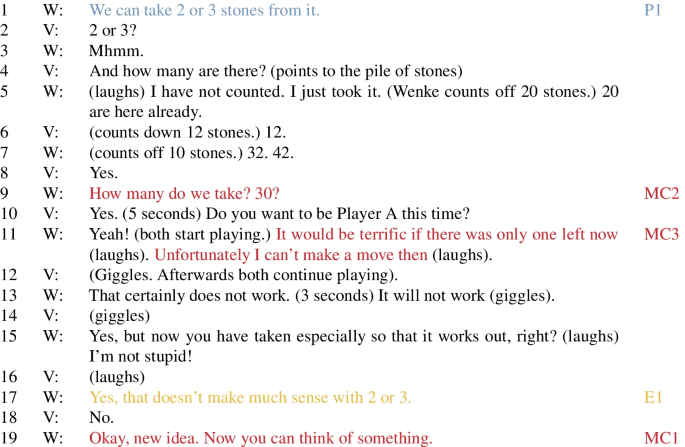
Overall, in this process, Valerie and Wenke show noticeably insecurities. This can be seen through the numerous occurrences of laughter. Nevertheless, they also show metacognitive behavior — although less frequently than Tino and Ulrich. Like Tino and Ulrich, they realize during the game that an inevitable situation could occur. In Turn 17, Wenke also evaluates this, but they see this situation as a reason to reject the posed game.
Research Objective (1): Development of a framework for identifying problem-posing-specific aspects of metacognitive behavior (i.e. planning , monitoring & control , and evaluating ) in pre-service teachers’ problem-posing processes

Research Objective (2): Application of the framework developed in (1) as a proof of concept to make differences regarding metacognitive behavior in problem-posing processes visible

The perspective on the metacognitive behavior of both pairs reveals differences between the processes. Since in the selected excerpts the posed problems are rather identical, no differences would have been attested by looking only at the products. It also matters that Tino and Ulrich have a better understanding of the posed problem by suggesting a solution strategy as well as their motivational beliefs. However, the task did not explicitly ask for solving the posed problems. Tino’s and Ulrich’s impetus to do so independently may attest motivational beliefs that are certainly relevant in the context of metacognitive behavior.
The aim of the present explorative study was to investigate metacognitive behavior in problem-posing processes, which has been widely disregarded in problem-posing research. Analyses of 32 problem-posing processes of pre-service teacher students were conducted to identify metacognitive behaviors of planning , monitoring & control , and evaluating . Tables 3 , 4 , and 5 summarize these inductively developed categories of problem-posing-specific aspects of metacognitive behavior. In addition, two transcript excerpts were analyzed using the previously developed codes as a proof of concept to make differences regarding metacognitive behavior in problem-posing processes visible. Although, in both transcript excerpts, the product in terms of the posed problem is almost identical, the metacognitive behavior, as the analysis has shown, differs. The identified problem-posing-specific aspects of metacognitive behaviors enabled the disclosure of these differences. In addition, the analyses have shown that the consideration of metacognitive behavior allows a tentative assessment of the quality of the activity in general. This assessment is a new perspective on problem-posing processes.
Limitations of this study lie especially in the method of analyzing statements of pairs of students in a video-based content analysis to assess metacognitive behavior. As Goos et al. ( 2002 ) pointed out “student-student interactions could either help or hinder metacognitive decision making during paired problem solving, depending on students’ flexibility in sharing metacognitive roles” (p. 197). This approach can be enriched in future studies by using stimulated recall interviews. Eye-tracking can serve as a potential stimulus of such interviews. Individual interviews with a thinking-aloud approach were not used because thinking aloud, unpracticed, can interfere with the natural flow of such processes and, in particular, affect metacognitive activities (McKeown & Gentilucci, 2007 ), which could distort the analyses.
The framework developed in this study provides numerous opportunities for follow-up research on problem posing. As this study is based on problem-posing processes of student teachers, it would be of interest to see if additional problem-posing-specific aspects of metacognitive behaviors can be identified in a sample of, for example, pupils or expert problem posers. A larger analysis could also address the question of which metacognitive behaviors of planning, monitoring & control, and evaluating are particularly prevalent. As in research on problem solving, a comparison between metacognitive behaviors of experts and novices could reveal whether metacognitive behavior related to successful problem posing or whether there are substantial differences in metacognitive behavior in general. The data of this study was collected through structured problem-posing situations. Future studies could address the question of whether there are different metacognitive behaviors in unstructured situations or whether there are significant differences in the frequency of the different metacognitive behaviors between structured and unstructured problem-posing situations. Often, the ability to pose problems is measured by analyzing the products of a problem-posing process (Bonotto, 2013 ; Singer et al., 2017 ; Van Harpen & Sriraman, 2013 ). The analysis of metacognitive behavior could be used to assess the quality of problem posing on a process-oriented level. It is likely that there is a strong correlation between the products and the processes of problem posing. However, there may also be high-quality processes by means of metacognitive behavior, but whose products attest lower quality because few and non-original problems were posed. Neglected in this study was the metacognitive facet knowledge about cognition. The importance of this facet of metacognition could also be the focus of future studies. Furthermore, metacognitive processes should be considered in addition to motivational beliefs (Zimmerman & Moylan, 2009 ). Those beliefs could certainly enrich the comparison of the two processes. Tino and Ulrich exhibit numerous behaviors indicative of their motivational beliefs. Even before the analyzed extract, Tino and Ulrich have numerous ideas about the Nim game. In turn 5, Ulrich shows that he would like to deal with the different ideas one after the other, presumably in order to do sufficient justice to all of them. Also, their numerous evaluative statements regarding their ideas (Turn 13; 17; 18; 25) as well as thinking their ideas further (Turn 20; 21; 26; 27) are an expression of intrinsic interest in posing new problems. In Valerie and Wenke’s process, such behaviors and especially the positive reference to the activity of problem posing are largely absent. Their laughter rather speaks for a general insecurity and a tendency towards low interest in problem posing. Future studies could focus more on this interplay between metacognitive behavior and motivational beliefs.
For teaching in school and university settings, these study’s findings can be used to plan problem-posing activities in the classroom (cf. Kontorovich et al., 2012 ). For example, students could be encouraged to engage in metacognitive behavior during problem posing through appropriate construction of the problem-posing situation by the teacher. The teacher could ask the students to solve their posed problems or they could encourage reflection on the tasks by requiring students to pose tasks of varying difficulty for their classmates.
This study provides a first, qualitative insight into metacognitive behavior in problem posing. We hope that the perspective of metacognition will stimulate further studies in the field of problem posing research to gain further insights.
The current version of this coding manual can be found at https://www.mathematik.uni-osnabrueck.de/fileadmin/didaktik/Projekte_KM/Kategoriensystem_EN.pdf .
Artzt, A. F., & Armour-Thomas, E. (1992). Development of a cognitive-metacognitive framework for protocol analysis of mathematical problem solving in small groups. Cognition and Instruction , 9 (2), 137–175. https://doi.org/10.1207/s1532690xci0902_3
Article Google Scholar
Artzt, A. F., & Armour-Thomas, E. (1997). Mathematical problem solving in small groups: Exploring the interplay of students’ metacognitive behaviors, perceptions, and ability levels. The Journal of Mathematical Behavior , 16 (1), 63–74. https://doi.org/10.1016/S0732-3123(97)90008-0
Baumanns, L., & Rott, B. (2021a). Developing a framework for characterizing problem-posing activities: A review. Research in Mathematics Education , 24 (1), 28–50. https://doi.org/10.1080/14794802.2021.1897036
Baumanns, L., & Rott, B. (2021b). Rethinking problem-posing situations: A review. Investigations in Mathematics Leaning , 13 (2), 59–76. https://doi.org/10.1080/19477503.2020.1841501
Baumanns, L., & Rott, B. (2022). The process of problem posing: Development of a descriptive phase model of problem posing. Educational Studies in Mathematics , 110 , 251–269. https://doi.org/10.1007/s10649-021-10136-y
Bonotto, C. (2013). Artifacts as sources for problem-posing activities. Educational Studies in Mathematics , 83 (1), 37–55. https://doi.org/10.1007/s10649-012-9441-7
Brown, S. I., & Walter, M. I. (1983). The art of problem posing . Franklin Institute Press.
Brown, S. I., & Walter, M. I. (2005). The art of problem posing (3rd edn.). Lawrence Erlbaum Associates.
Butts, T. (1980). Posing problems properly. In S. Krulik & R. E. Reys (Eds.), Problem solving in school mathematics (pp. 23–33) . NCTM.
Cai, J., & Hwang, S (2020). Learning to teach through mathematical problem posing: Theoretical considerations, methodology, and directions for future research. International Journal of Educational Research , 102 , 1–8. https://doi.org/10.1016/j.ijer.2019.01.001
Cai, J., Hwang, S., Jiang, C., & Silber, S (2015). Problem-posing research in mathematics education: Some answered and unanswered questions. In F. M. Singer, N. F. Ellerton, & J. Cai (Eds.), Mathematical problem posing. from research to effective practice (pp. 3–34). Springer.
Cai, J., & Leikin, R. (2020). Affect in mathematical problem posing: Conceptualization, advances, and future directions for research. Educational Studies in Mathematics , 105 , 287–301. https://doi.org/10.1007/s10649-020-10008-x
Carrillo, J., & Cruz, J (2016). Problem-posing and questioning: Two tools to help solve problems. In P. Felmer, E. Pehkonen, & J. Kilpatrick (Eds.), Posing and Solving Mathematical. Problems Advances and New Perspectives (pp. 24–36) . Springer.
Christou, C., Mousoulides, N., Pittalis, M., Pitta-Pantazi, D., & Sriraman, B. (2005). An empirical taxonomy of problem posing processes. ZDM – Mathematics Education , 37 (3), 149–158. https://doi.org/10.1007/s11858-005-0004-6
Cohors-Fresenborg, E., & Kaune, C. (2007). Modelling classroom discussions and categorizing discursive and metacognitive activities. In D. Pitta-Pantazi & G. Philippou (Eds.), Proceedings of the Fifth Congress of the European Society for Research in Mathematics Education (pp. 1180–1189). ERME.
Cohors-Fresenborg, E., Kramer, S., Pundsack, F., Sjuts, J., & Sommer, N. (2010). The role of metacognitive monitoring in explaining differences in mathematics achievement. ZDM – Mathematics Education , 42 (2), 231–244. https://doi.org/10.1007/s11858-010-0237-x
Crespo, S. (2003). Learning to pose mathematical problems: Exploring changes in preservice teachers’ practices. Educational Studies in Mathematics , 52 (3), 243–270. https://doi.org/10.1023/A:1024364304664
Cross, D. R., & Paris, S. G. (1988). Developmental and instructional analyses of children’s metacognition and reading comprehension. Journal of Educational Psychology , 80 (2), 131–142. https://doi.org/10.1037/0022-0663.80.2.131
Cruz, M. (2006). A mathematical problem-formulating strategy. International Journal for Mathematics Teaching and Learning , 79–90.
Desoete, A., Roeyers, H., & Buysse, A. (2001). Metacognition and mathematical problem solving in grade 3. Journal of Learning Disabilities , 34 (5), 435–447. https://doi.org/10.1177/002221940103400505
Ellerton, N. F., Singer, F. M., & Cai, J. (2015). Problem posing in mathematics: Reflecting on the past, energizing the present, and foreshadowing the future. In F. M. Singer, N. F. Ellerton, & J. Cai (Eds.), Mathematical Problem Posing. From Research to Effective Practice (pp. 547–556). Springer.
Erkan, B., & Kar, T. (2022). Pre-service mathematics teachers’ problem-formulation processes: Development of the revised active learning framework. Journal of Mathematical Behavior , 65 , 100918. https://doi.org/10.1016/j.jmathb.2021.100918
Flavell, J. H. (1979). Metacognition and cognitive monitoring. A new area of cognitive-developmental inquiry. American Psychologist , 34 (10), 906–911.
Flick, U. (2007). Managing quality in qualitative research . Sage.
Garofalo, J., & Lester, F. K. (1985). Metacognition, cognitive monitoring, and mathematical performance. Journal for Research in Mathematics Education , 16 (3), 163–176.
Ghasempour, A. Z., Baka, M. N., & Jahanshahloo, G. R. (2013). Mathematical problem posing and metacognition: A theoretical framework. International Journal of Pedagogical Innovations , 1 (2), 63–68.
Goos, M., Galbraith, P., & Renshaw, P. A. (2002). Socially mediated metacognition: Creating collaborative zomes of proximal development in small group problem solving. Educational Studies in Mathematics , 49 , 192–223. https://doi.org/10.1023/A:1016209010120
Hadamard, J. (1945). The psychology of invention in the mathematical field . Dover Publications.
Halmos, P. R. (1980). The heart of mathematics. The American Mathematical Monthly , 87 (7), 519–524.
Johnson, D., & Johnson, R. (1999). Learning together and alone: Cooperative, competitive and individualistic learning . Prentice Hall.
Joklitschke, J., Baumanns, L., & Rott, B. (2019). The intersection of problem posing and creativity: A review. In M. Nolte (Ed.), Including the highly gifted and creative students – Current ideas and future directions. Proceedings of the 11th International Conference on Mathematical Creativity and Giftedness (MCG11) (pp. 59–67). WTM.
Kaune, C. (2006). Reflection and metacognition in mathematics education – Tools for the improvement of teaching quality. ZDM – Mathematics Education , 38 (4), 350–360. https://doi.org/10.1007/BF02652795
Kilpatrick, J. (1987). Problem formulating: Where do good problems come from? In A. H. Schoenfeld & J. Kilpatrick (Eds.) Cognitive science and mathematics education (pp. 123–147). Lawrence Erlbaum Associates.
Kim, Y. R., Park, M. S., Moore, T. J., & Varma, S. (2013). Multiple levels of metacognition and their elicitation through complex problem-solving tasks. The Journal of Mathematical Behavior , 37 , 337–396. https://doi.org/10.1016/j.jmathb.2013.04.002
Koichu, B., & Kontorovich, I. (2013). Dissecting success stories on mathematical problem posing: A case of the billiard task. Educational Studies in Mathematics , 83 (1), 71–86. https://doi.org/10.1007/s11251-012-9254-1
Kontorovich, I., Koichu, B., Leikin, R., & Berman, A. (2012). An exploratory framework for handling the complexity of mathematical problem posing in small groups. The Journal of Mathematical Behavior, 31 (1), 149–161. https://doi.org/10.1016/j.jmathb.2011.11.002
Kuhn, D., & Dean, D. (2004). Metacognition: A bridge between cognitive psychology and educational practice. Theory into Practice , 43 (4), 268–273. https://doi.org/10.1207/s15430421tip4304_4
Kuzle, A. (2013). Patterns of metacognitive behavior during mathematics problem-solving in a dynamic geometry environment. International Electronic Journal of Mathematics Education , 8 (1), 20–40.
Lang, S. (1989). Faszination Mathematik – Ein Wissenschaftler stellt sich der Öffentlichkeit [The fascination of mathematics – A scientist faces the public]. Vieweg.
Lee, S.-Y. (2021). Research status of mathematical problem posing in mathematics education journals. International Journal of Science and Mathematics Education, 19 , 1677–1693. https://doi.org/10.1007/s10763-020-10128-z
Mayring, P. (2000). Qualitative content analysis. Forum: Qualitative Social Research , 1 (2), Art. 20.
McKeown, R. G., & Gentilucci, J. L. (2007). Think-aloud strategy: Metacognitive development and monitoring comprehension in the middle school second-language classroom. Journal of Adolescent & Adult Literacy , 51 (2), 136–147. https://doi.org/10.1598/JAAL.51.2.5
Mevarech, Z., & Fridkin, S. (2006). The effects of IMPROVE on mathematical knowledge, mathematical reasoning and meta-cognition. Metacognition and Learning , 1 (1), 85–97. https://doi.org/10.1007/s11409-006-6584-x
Özsoy, G., & Ataman, A. (2009). The effect of metacognitive strategy training on mathematical problem solving achievement. International Electronic Journal of Elementary Education , 1 (2), 67–82.
Google Scholar
Pelczer, I., & Gamboa, F. (2009). Problem posing: Comparison between experts and novices. In M. Tzekaki, M. Kaldrimidou, & H. Sakonidis (Eds.), Proceedings of the 33 Conference of the International Group for the Psychology of Mathematics Education (Vol. 4, pp. 353–360). PME.
Pintrich, P. R. (2000). The role of goal orientation in self-regulated learning. In M. Boekaerts, P. R. Pintrich, & M. Zeidner (Eds.), Handbook of self-regulation (pp. 451–529). Academic Press.
Pintrich, P. R. (2002). The role of metacognitive knowledge in learning, teaching, and assessing. Theory Into Practice , 41 (4), 219–225. https://doi.org/10.1207/s15430421tip4104_3
Pólya, G. (1945). How to solve it . University Press.
Rott, B. (2013). Process regulation in the problem-solving processes of fifth graders. CEPS Journal , 3 (4), 25–39. https://doi.org/10.26529/cepsj.221
Rott, B. (2014). Mathematische Problembearbeitungsprozesse von Fünftklässlern – Entwicklung eines deskriptiven Phasenmodells [Mathematical problem-solving processes of fifth graders - development of a descriptive phase model]. Journal für Mathematik-Didaktik , 35 (2), 251–282. https://doi.org/10.1007/s13138-014-0069-2
Ruthven, K. (2020). Problematising learning to teach through mathematical problem posing. International Journal of Educational Research , 102 , 1–7. https://doi.org/10.1016/j.ijer.2019.07.004
Schneider, W., & Artelt, C (2010). Metacognition and mathematics education. ZDM – Mathematics Education , 42 (2), 149–161. https://doi.org/10.1007/s11858-010-0240-2
Schoenfeld, A. H. (1985a). Making sense of “out loud” problem-solving protocols. The Journal of Mathematical Behavior , 4 , 171–191.
Schoenfeld, A. H. (1985b). Mathematical problem solving . Academic Press.
Schoenfeld, A. H. (1987). What’s all the fuss about metacognition? In A. H. Schoenfeld (Ed.) Cognitive science and mathematics education (pp. 189–215). Lawrence Erlbaum Associates.
Schoenfeld, A. H. (1992). Learning to think mathematically: Problem solving, metacognition and sense-making in mathematics. In D. Grouws (Ed.), Handbook of research on mathematics teaching and learning (pp. 334–370). MacMillan.
Schraw, G., & Moshman, D. (1995). Metacognitive theories. Educational Psychology Review , 7 (4), 351–371. https://doi.org/10.1007/BF02212307
Schupp, H. (2002). Thema mit Variationen. Aufgabenvariationen im Mathematikunterricht [Theme with variations. Task variations in mathematics lessons] . Franzbecker.
Silver, E. A. (1994). On mathematical problem posing. For the Learning of Mathematics , 14 (1), 19–28.
Silver, E. A. (1995). The nature and use of open problems in mathematics education: Mathematical and pedagogical perspectives. ZDM – Mathematics Education , 27 (2), 67–72.
Silver, E. A. (1997). Fostering creativity through instruction rich in mathematical problem solving and problem posing. ZDM – Mathematics Education , 29 (3), 75–80. https://doi.org/10.1007/s11858-997-0003-x
Silver, E. A. (2013). Problem-posing research in mathematics education: Looking back, looking around, and looking ahead. Educational Studies in Mathematics , 83 (1), 157–162. https://doi.org/10.1007/s10649-013-9477-3
Singer, F. M., & Voica, C (2015). Is problem posing a tool for identifying and developing mathematical creativity? In F. M. Singer, N. F. Ellerton, & J. Cai (Eds.), Mathematical Problem Posing. From Research to Effective Practice (pp. 141–174). Springer.
Singer, F. M., Voica, C., & Pelczer, I. (2017). Cognitive styles in posing geometry problems: Implications for assessment of mathematical creativity. ZDM – Mathematics Education , 49 (1), 37–52. https://doi.org/10.1007/s11858-016-0820-x
Stoyanova, E. (1997). Extending and exploring students’ problem solving via problem posing (Doctoral dissertation). Edith Cowan University.
Stoyanova, E. (1999). Extending students’ problem solving via problem posing. Australian Mathematics Teacher , 55 (3), 29–35.
Stoyanova, E., & Ellerton, N. F (1996). A framework for research into students’ problem posing in school mathematics. In P. C. Clarkson (Ed.), Technology in mathematics education (pp. 518–525). Mathematics Education Research Group of Australasia.
Tichá, M, & Hošpesová, A (2013). Developing teachers’ subject didactic competence through problem posing. Educational Studies in Mathematics , 83 (1), 133–143. https://doi.org/10.1007/s10649-012-9455-1
Van der Stel, M., Veenman, M. V. J., Deelen, K., & Haenen, J. (2010). The increasing role of metacognitive skills in math: A cross-sectional study from a developmental perspective. ZDM – Mathematics Education , 42 (2), 219–229.
Van Harpen, X., & Sriraman, B. (2013). Creativity and mathematical problem posing: An analysis of high school students’ mathematical problem posing in China and the USA. Educational Studies in Mathematics , 82 (2), 201–221. https://doi.org/10.1007/s10649-012-9419-5
Veenman, M. V. J., Van Hout-Wolters, B. H. A. M., & Afflerbach, P. (2006). Metacognition and learning: Conceptual and methodological considerations. Metacognition Learning , 1 , 3–14. https://doi.org/10.1007/s11409-006-6893-0
Voica, C., Singer, F. M., & Stan, E. (2020). How are motivation and self-efficacy interacting in problem-solving and problem-posing?. Educational Studies in Mathematics , 105 (3), 487–517. https://doi.org/10.1007/s10649-020-10005-0
Whitebread, D., Coltman, P., Pasternak, D. P., Sangster, C., Grau, V., Bingham, S., Almeqdad, Q., & Demetriou, D. (2009). The development of two observational tools for assessing metacognition and self-regulated learning in young children. Metacognition and Learning , 4 (1), 63–85. https://doi.org/10.1007/s11409-008-9033-1
Yimer, A., & Ellerton, N. F. (2010). A five-phase model for mathematical problem solving: Identifying synergies in pre-service-teachers’ metacognitive and cognitive actions. ZDM – Mathematics Education , 42 (2), 245–261. https://doi.org/10.1007/s11858-009-0223-3
Yuan, X., & Sriraman, B. (2011). An exploratory study of relationships between students’ creativity and mathematical problem-posing abilities. In B. Sriraman, K. H. Lee, & X. Yuan (Eds.), The Elements of Creativity and Giftedness in Mathematics (pp. 5–28). Sense Publishers.
Zimmerman, B. J., & Moylan, A. R. (2009). Self-regulation. In J. Hacker, J. Douglas, A. C. Graesser, & B. J. Zimmerman (Eds.), Where metacognition and motivation intersect (pp. 299–315). Routledge.
Download references
Open Access funding enabled and organized by Projekt DEAL.
Author information
Authors and affiliations.
Institute of Mathematics Education, University of Cologne, Gronewaldstr. 2, D-50931, Köln, Germany
Lukas Baumanns & Benjamin Rott
You can also search for this author in PubMed Google Scholar
Corresponding author
Correspondence to Lukas Baumanns .
Ethics declarations
Ethical approval.
Informed consent was obtained from all participants included in the study.
Data handling and analysis followed the rights of the General Data Protection Regulation (Datenschutz-Grundverordnung - DSGVO).
Additional information
Publisher’s note.
Springer Nature remains neutral with regard to jurisdictional claims in published maps and institutional affiliations.
Rights and permissions
Open Access This article is licensed under a Creative Commons Attribution 4.0 International License, which permits use, sharing, adaptation, distribution and reproduction in any medium or format, as long as you give appropriate credit to the original author(s) and the source, provide a link to the Creative Commons licence, and indicate if changes were made. The images or other third party material in this article are included in the article's Creative Commons licence, unless indicated otherwise in a credit line to the material. If material is not included in the article's Creative Commons licence and your intended use is not permitted by statutory regulation or exceeds the permitted use, you will need to obtain permission directly from the copyright holder. To view a copy of this licence, visit http://creativecommons.org/licenses/by/4.0/ .
Reprints and permissions
About this article
Baumanns, L., Rott, B. Identifying Metacognitive Behavior in Problem-Posing Processes. Int J of Sci and Math Educ 21 , 1381–1406 (2023). https://doi.org/10.1007/s10763-022-10297-z
Download citation
Received : 17 February 2021
Accepted : 12 June 2022
Published : 05 September 2022
Issue Date : June 2023
DOI : https://doi.org/10.1007/s10763-022-10297-z
Share this article
Anyone you share the following link with will be able to read this content:
Sorry, a shareable link is not currently available for this article.
Provided by the Springer Nature SharedIt content-sharing initiative
- Problem posing
- Find a journal
- Publish with us
- Track your research

IMAGES
COMMENTS
1. Introduction. Metacognition is a multi-faceted phenomenon that involves both the awareness and regulation of one's cognitions (Flavell 1979).Past research has shown that metacognitive regulation, or the skills learners use to manage their cognitions, is positively related to effective problem-solving (Berardi-Coletta et al. 1995), transfer (Lin and Lehman 1999), and self-regulated ...
reasoning and problem-solving (Ader, 2019). Metacognitive skills play a critical role in monitoring and. regulating cognitive processes (Chan & Mansoor, 2007) and help stude nts to understand when ...
Metacognition is defined as "thinking about thinking" or the ability to monitor and control one's cognitive processes 1 and plays an important role in learning and education 2,3,4.For ...
Metacognition is the practice of being aware of one's own thinking. Some scholars refer to it as "thinking about thinking.". Fogarty and Pete give a great everyday example of metacognition ...
To solve problems, there is a need not only for carrying out the stages of problem-solving but also for following, regulating, and controlling these stages consciously. Therefore, metacognition is an essential element of the problem-solving process (Schoenfeld, 2005). Although various studies have revealed the positive relationship
Metacognition is, put simply, thinking about one's thinking. More precisely, it refers to the processes used to plan, monitor, and assess one's understanding and performance. Metacognition includes a critical awareness of a) one's thinking and learning and b) oneself as a thinker and learner. Initially studied for its development in young ...
Even when there is evidence of the relation between metacognitive processes and critical thinking, there are still few initiatives which seek to clarify which process determines which other one, or whether there is interdependence between both. ... " Metacognitive aspects of problem solving," in The Nature of Intelligence. ed. Resnik L. B ...
develop their metacognitive processes. Fogarty (1994) suggests that metacognition is a process that spans three distinct phases, and that, to be successful thinkers, students must do the follow-ing: 1. Develop a . plan. before approaching a learning task, such as reading for comprehension or solv-ing a math problem. 2. Monitor
Metacognition is the ability of learners to monitor and control their cognitive process (Young & Fry, 2008) for improving academic achievements (Tanner, 2012; van der Stel & Veenman, 2014), fostering reading and comprehension skills (Reeve & Brown, 1984), advancing critical thinking skills (Ku & Ho, 2010) and enhancing problem solving and ...
Metacognition, Problem, and Problem-Solving . Metacognition, problem, and problem-solving processes are of great importance and are the basic skills that should be acquired in mathematics education (Artzt and Armor-Thomas, 1992; Lucangeli et al., 2019; Jacobse and Harskamp, 2012; Posamentier and Krulik, 2008; Smith and Mancy, 2018).
Metacognition in Collaborative Problem Solving. CPS is a joint activity in which multiple individuals combine their resources, skills, and efforts to transform a problem state into a desired state (Roschelle & Teasley, 1995).CPS offers a rich source to support or prompt metacognition in cognitive and social processes.
Metacognition was measured using a. -. metacognitive knowledge interview (declarative metacognition) and a metacognitive skills observational scale (procedural), both in the context of a problem-solving puzzle task. Executive function was assessed with the Head Toes Knees Shoulder measure and motivation was operationalized as persistence ...
Problem orientation is defined as "a metacognitive process involving the operation of a set of relatively stable cognitive-emotional schemas that reflect a person's general beliefs, appraisals, and feelings about problems in living, as well as his or her own problem-solving ability" (D'Zurilla et al., 2004, p. 14).
Metacognition comprises both the ability to be aware of one's cognitive processes (metacognitive knowledge) and to regulate them (metacognitive control). ... subjects are asked to verbalise their thoughts while performing a problem-solving task 80. Each of these instruments can be used to study meta-knowledge and meta-control. For instance, ...
The metacognition in creative problem-solving (MCPS) scale was introduced to self-assess metacognitive skills during the creative problem-solving process.. The MCPS scale is designed to capture the engagement in planning, monitoring, regulation, and evaluation in creative problem-solving, being more suitable for problem-solving research than other instruments focusing on learning-specific ...
While the link between metacognitive skills and the creativity of outcomes was established only recently, further exploration is required to understand their interplay in complex problem-solving. This explanatory sequential mixed-methods study investigated the role of metacognition in solving two ill-defined problem-solving tasks: an ...
Metacognition is an awareness of one's thought processes and an understanding of the patterns behind them. The term comes from the root word meta, meaning "beyond", or "on top of". [1] Metacognition can take many forms, such as reflecting on one's ways of thinking, and knowing when and how oneself and others use particular strategies for problem-solving.
The strategies suggested by Montague (1992) for problem solving according to metacognitive process consist of seven stages: 1) study the problem to understand it, 2) paraphrase the problem (in your own words), 3) visualize the problem, 4) hypothesize (a program to solve the problem), 5) estimate (predict the problem), 6) compute (computational ...
Fogarty (1994) suggests that Metacognition is a process that spans three distinct phases, and that, to be successful thinkers, students must do the following: Develop a plan before approaching a learning task, such as reading for comprehension or solving a math problem. Monitor their understanding; use "fix-up" strategies when meaning ...
In this study, the first three of the six metacognitive processes are identified and clustered as. the problem solving task-related metacognition. On the other hand, the next three are grouped ...
Students, who initially lacked structured problem-solving skills, found that scaffolding helped them to slow down metacognitive processes that would otherwise be rushed through or engaged with on a surface level. Students' resistance to the scaffold was due to fear of making a mistake or viewing the scaffold as requiring extra time and effort.
We did this in the context of a problem-solving task based on literature indicating that individuals tend to display higher levels of metacognitive and executive processes while problem-solving (Antonietti et al. 2000; Aşık and Erktin 2019). We had two overarching aims: 1) to examine early years Mc and compare results across different ...
Participants identified goal setting, planning, problem-solving, and checking/reflection as the most important elements of CO-OP's metacognitive approach. A hallmark of CO-OP is the global strategy "goal-plan-do-check," which is taught and practiced iteratively, empowering patients to use a structured, metacognitive approach to problem ...
This scaffolding or progressive problem-solving approach is a critical part of developing expertise. It is the gaining of experience for both content but process that is important. Experts are more metacognitively aware than novices because the progression of problem solving requires the cognitive and metacognitive processes. 64
As a process, problem-solving involves a series of steps for finding solutions to questions or concerns that arise throughout life. The importance of problem-solving for managers. Managers deal with problems regularly, whether supervising a staff of two or 100. Workplaces can benefit in several ways when people solve problems quickly and ...
The results indicated that in at least one of six problem-solving tasks ChatGPT provided a mathematically incorrect answer. The processes appearing in ChatGPT texts, aiming at developing students' understanding of mathematical concepts, included verbal, mental, existential, relational and behavioural processes but no material processes.
Insights into the process of mathematical problem posing is a central concern in mathematics education research. However, little is known about regulative or metacognitive behaviors that are essential to understanding this process. In this study, we investigate metacognitive behavior in problem posing. We aim at (1) identifying problem-posing-specific metacognitive behaviors and (2) applying ...
He asked candidates about their toughest problems and decision-making processes. This approach aimed to uncover genuine problem-solving abilities and authenticity, revealing that detailed ...